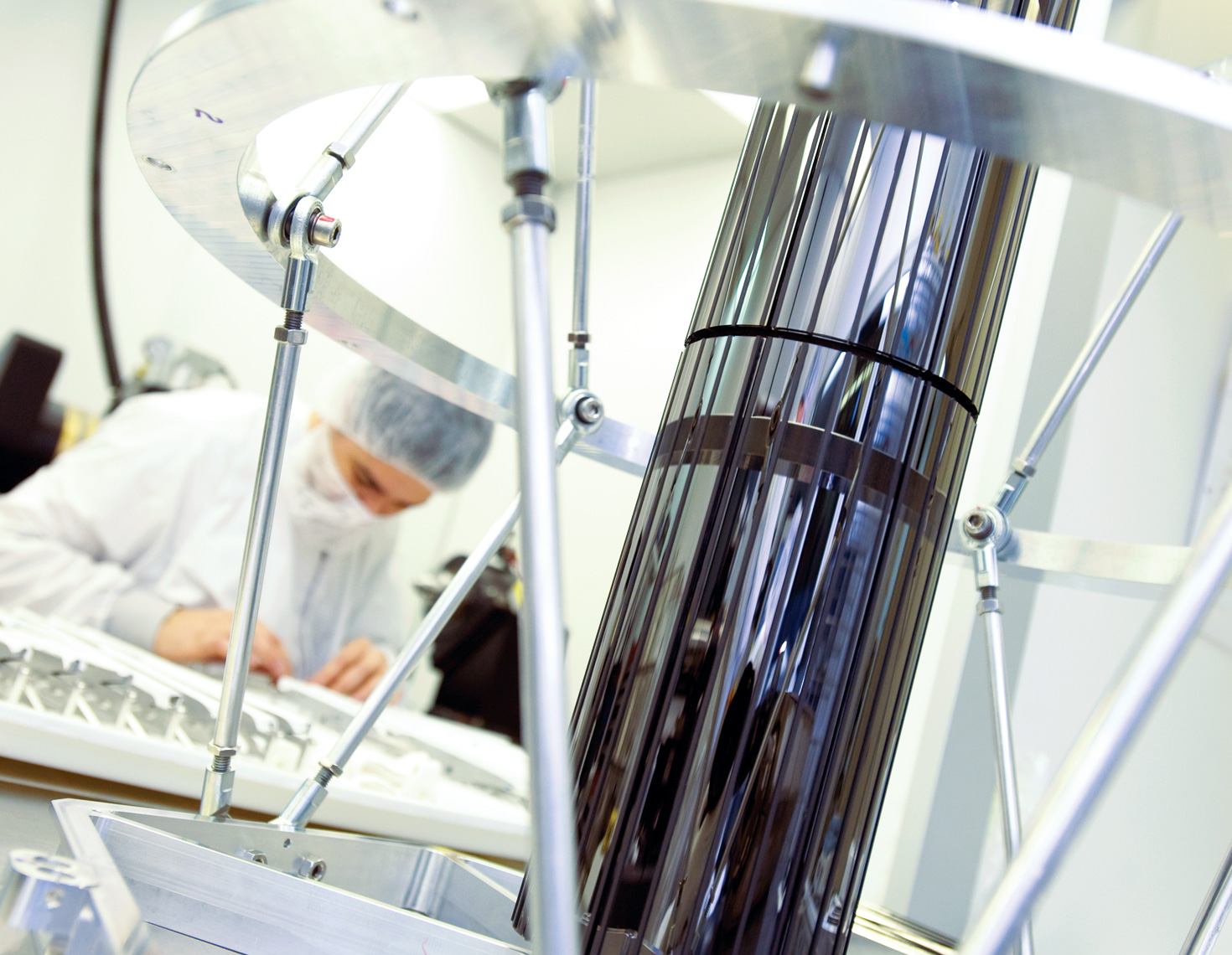
It may look like a polished artillery canister, but it’s as fragile as an eggshell. Peer inside and you’ll see 133 cylinders, all made of paper-thin glass. The cylinders are arranged concentrically so that, when viewed from either end, they resemble the rings of a halved onion.
Astronomers call this type of optic device a “light bucket,” because when it’s pointed at a star it will collect every bit of light offered. But this light bucket, designed by Columbia astrophysicist Chuck Hailey, is different from others. Most telescopes today use a dish-shaped mirror to collect light and to reflect the beams back, at slightly different angles, toward a focal point. When Hailey’s optic gets launched into space next year, as part of a new NASA satellite telescope, light beams will shoot in one end, through tiny spaces in between its glass cylinders, and exit out the back. In the process, something unprecedented will occur: Extremely high energy light beams known as hard X-rays, which would penetrate straight through the components of any other telescope, will get reflected slightly, and set on paths to converge at a focal point 30 feet away — at the far end of the satellite, called the Nuclear Spectroscopic Telescope Array (NuSTAR). There, digital sensors will receive the X-rays and transmit their properties down to scientists who will generate computer images of the stars from which the X-rays came.
This elegant instrument is the first ever capable of focusing hard X-rays. (Hard X-rays vibrate at higher frequencies than the so-called soft X-rays used in medical imaging, which in turn are “stronger” than visible light.) It is a technological centerpiece of NuSTAR, which is scheduled to launch into Earth’s orbit in late 2011.
“We want to focus hard X-rays because some celestial phenomena emit no other type of light,” says Hailey, who oversees a team of 15 scientists and engineers building the optic at Columbia’s Nevis Laboratories in Irvington, New York. “Neutron stars, for instance, can produce flares so hot that we can’t see them.”
The NuSTAR telescope should lead to spectacular discoveries. For starters, scientists will gain a deeper understanding of the extreme physics that occur on ultrahot bodies like neutron stars, which can be invisible altogether. They’ll also get a peek inside gigantic clouds of space dust. The center of our own galaxy, for example, is shrouded by gas and debris generated by the deaths of many stars. Only hard X-rays (and even stronger gamma rays) typically escape the maelstrom that lingers around collapsed stars for billions of years. To glimpse a neutron star inside this region, and to track its movement, will be of great value to scientists. Most notably, it will help them spot black holes, which are identifiable by the circular patterns that celestial bodies chart around them.
“I can’t wait to see into the center of the Milky Way,” says Hailey ’83GSAS, who earned his PhD in physics at Columbia. “The galactic center is the real zoo. That’s where the black holes have to be, because black holes are born where stars collapse. Only a couple of dozen black holes have ever been discovered within our galaxy. This is our chance to discover literally thousands of them and to really study their properties. We’re about to lift the curtain.”
Black-hole hunters
Hailey’s invention capitalizes on a curious characteristic of hard X-rays: They lose their penetrative power when they hit a flat surface at a very shallow angle, in which case they’ll reflect off the surface, like a rock skipped off of water. NuSTAR’s glass cylinders will be positioned nearly parallel to incoming X-rays. The cylinders are skinnier at their tail ends, forcing X-rays to glance off their inner surfaces, not once but twice, reflecting the X-rays inward about a quarter degree each time.
“X-rays have very limited reflective properties,” says Hailey, who is Columbia’s Pupin Professor of Physics, “but they’re made of photons, and just like any photons, they’ll bounce off a mirror. You just have to catch them on their sweet spot.”
Astronomers have been using cylindrical mirrors to focus high-energy wavelengths of light since the late 1960s. But no telescope has ever achieved the tiny reflection angles necessary to focus hard X-rays. NASA’s Chandra X-ray Observatory, for example, employs four cylinders nested together less tightly than are NuSTAR’s, enabling Chandra to focus light only in the soft X-ray spectrum. NuSTAR is unique also for having more cylindrical shells than any existing telescope. Its 133 layers of glass would cover a Little League baseball diamond if spread out on the ground. That represents an exposure area three times as large as Chandra’s and it will enable NuSTAR to produce images of stars that emit even the faintest trace of hard X-rays.
The drawback of using a giant aperture is that resultant images won’t have exquisite resolution. Every photographer understands this trade-off: if you’re shooting in low light, you must sacrifice some detail to get any picture at all. But this fits the exploratory nature of NuSTAR’s mission. Whereas NASA launched its Chandra and Hubble observatories in the 1990s in part to make highly detailed pictures of phenomena already observed — which requires moderating the amount of light entering a telescope at any moment — NuSTAR’s goal is to make useful, but not necessarily beautiful, images of celestial objects never seen before.
Hailey and his NuSTAR colleagues, who include physicists from the California Institute of Technology and Stanford University, view themselves as pioneers exploring a new band of the light spectrum, identifying phenomena that future X-ray missions will observe more carefully. (Their project, as part of NASA’s Small Explorer class of missions, has a budget cap of about $110 million; large NASA satellites like Chandra and Hubble cost 10 to 20 times as much.) “We won’t be making sharply focused pictures like the big dogs,” says Hailey. “For us, the name of the game is to be the first into the hard X-ray band, to pluck the low-hanging fruit in terms of discoveries, and to demonstrate that our method for focusing hard X-rays works.”
Glass onion
Hailey was chosen to oversee NuSTAR’s optics team in part because of improvements he’s made to the way cylindrical mirrors are manufactured: First, thin sheets of glass similar to the glass used in computer screens and cellular phone displays are cut into long strips, heated in an oven, and melted into concave molds. Next, the curved glass pieces are coated with a metal film, which makes them reflective. Technicians then assemble the glass pieces into concentric cylinders, which are separated one layer from the next by tiny graphite spacers whose thicknesses are determined — to the nearest micron — by a computer-driven grinder wheel.
“There’s no way you could get 133 layers on this thing if you were determining the spaces between each shell by hand, which is the way this used to work,” says Todd Decker, a mechanical engineer at Columbia’s Nevis Laboratories. “Every tiny inaccuracy would add up and become huge. Even with the computer’s help, this work isn’t for the faint of heart.”
Perfecting the process took years. Hailey tried hundreds of epoxies before finding one suitable for gluing together the glass strips. “It had to be able to withstand temperature changes in space, even when applied in a layer one-micron thin,” he says. “I’m not joking when I say that I’m now considered one of the world’s top experts on epoxy. This was never my intention, and some of my physics colleagues think it’s pretty funny. But it’s what the work demanded.”
The assembly of mirror encasements should be finished by this fall. Hailey’s team has already built two prototypes, each having about 10 cylinders. Researchers shoot hard X-rays at them every day to see how they perform. “So far, they’re perfect,” says Jason Koglin, a research scientist in the Columbia physics department. “But when we finish all 133 layers this summer, we’ll likely have to do some extremely difficult calibrations to make sure it focuses properly.”
This work all takes place in a brightly lit, dust-free room that Hailey constructed in a gymnasium-sized work space at Nevis Laboratories. The space once housed a large cyclotron particle collider and now resembles an airplane hangar. It’s cluttered with welding equipment, nitrogen tanks, scaffolding, and small cranes. Hailey strolled about this Metropolis-like facility recently and discussed the heritage of scientists, from Sir Isaac Newton to the late Columbia X-ray astronomy pioneer Robert Novick, who, in order to peer into the cosmos, picked up hammers and metal-working tools.
“If you want to do important work in astrophysics, you can’t spend all of your time thinking about neutron stars and black holes,” said Hailey. “You also have to think about electronics and mechanical engineering and optics and the physical properties of glass and epoxy. To get the data, you have to go into the garage.”