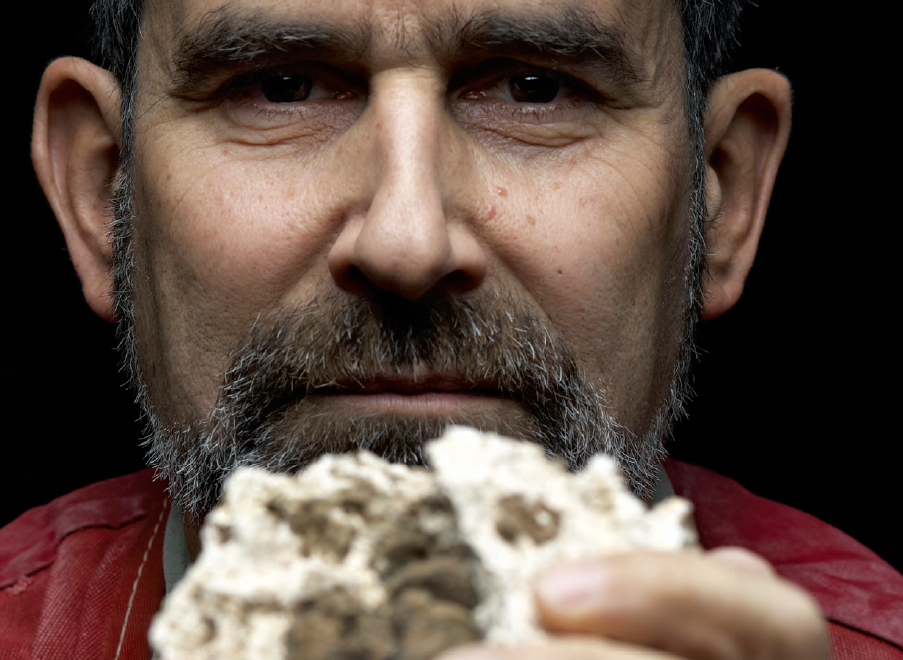
The first time Peter Kelemen visited the mountains of northern Oman, a craggy stretch of rock that pushed up through the earth’s surface ninety million years ago, he was disappointed to find cracks filled with carbonate, obscuring much of the landscape.
To him, it might as well have been Wite-Out, redacting millions of years of geological history. “When I would come to an outcrop full of all these white veins, I would turn and run the other way,” says Kelemen, a geochemist at Columbia’s Lamont-Doherty Earth Observatory.
It was 1994, and Kelemen had traveled to the desert Sultanate of Oman to study a coarse-grained, greenish rock called peridotite. The main constituent of the earth’s mantle, peridotite is normally hidden ten miles or more below ground. But a series of tectonic flukes under the Arabian Peninsula pushed a big chunk of mantle upward, forming the Hajar Mountains and making this one of the few places in the world where peridotite is accessible to scientists. Kelemen hoped that by studying the rock’s chemical composition he could reveal secrets about the extraordinarily hot, pressurized, and unstable subterranean world from which it came. The problem was that wherever peridotite had come into contact with carbon dioxide, the two had reacted to form a limestone-like solid. Much of the peridotite exposed to air or water was thus converted into a chalky substance, making it difficult for scientists to find clean samples.
“If our goal had been to study how carbon dioxide interacts with these rocks, this would have been great,” says Kelemen. “But we wanted to understand processes in the mantle. And the carbonate-filled cracks were obliterating the evidence.”
When Kelemen returned to Oman in 2007 for the first time in a few years, however, it was the carbonate he was looking for. Politicians and scientists in the United States had been buzzing about an ambitious plan to slow global warming by sucking carbon dioxide out of the air and storing it underground. One challenge was finding a safe place to lock it away.
Kelemen had a hunch his old nemesis could help. “These rocks suddenly seemed worth sampling,” he says. “Whereas once I bemoaned how they react with carbon dioxide, now I wanted to know: how much of the stuff could they take? And how fast?”
Mantle Pieces
Since the Industrial Revolution, the amount of carbon dioxide in Earth’s atmosphere has risen more than 40 percent, sealing in heat and wreaking havoc with our climate. The problem grows worse with every pound of fossil fuel we burn to fly, drive, watch television, or wash our clothes.
But what if there were a way to siphon that excess carbon dioxide right out of the air and stash it somewhere forever? The idea is known as carbon capture and storage, and while it may sound like wishful thinking, it’s won some prominent backers. Howard Herzog, a senior research engineer at the MIT Energy Initiative, says that developing a practical storage method is “critical for a secure, clean energy future.” President Obama has supported carbon capture since his first national campaign, and included in his 2009 federal stimulus package $3.4 billion for developing it.
To pull the carbon out of the air, researchers have devised some creative, if still speculative, solutions. Columbia physicist Klaus Lackner is working on giant synthetic trees that would absorb carbon dioxide like real trees do, but at a much greater rate. Harvard physicist David Keith has built a machine that sucks air through a thirty-foot-long chamber and extracts carbon dioxide using water laced with sodium hydroxide.
But what to do with the gas once it’s been captured? Some suggest compressing it into a liquid and injecting it into depleted oil wells, gas reservoirs, and other cavities underground. But critics warn that it could escape and cause damage to soil and water. Others suggest selling it to oil drillers, who use it to float oil out of the ground, or even selling it to carbonated-beverage manufacturers. But all the soda in the world isn’t going to use up the billions of tons of CO2 that scientists hope to pull from the air.
Kelemen is not a climate scientist, nor did he have much professional interest in global warming until a few years ago. But his experience working in Oman — since 1994 he has returned there regularly — gave him unique insight into the chemistry of carbon dioxide and rock. He knew that those streaks of carbonate that he had avoided occurred when magnesium and calcium in the mantle rock sucked up carbon dioxide from air and water, storing it, he says, “as an inert, harmless mineral.” He thought: what if peridotite could be made to do that on a massive scale? It might make the perfect storage facility.
Geologists had noticed that peridotite soaks up carbon dioxide long before Kelemen visited Oman. But most believed the process was impractical for large-scale sequestration. “I was told that people had thought about it and concluded that it was not practical because it was too slow,” Kelemen says. He was pretty sure they were wrong. “It didn’t match my intuition,” he says. “I had seen things that made me think the reactions could happen within hours.”
For example, pools of water that emerged from the rock and came in contact with air would develop a cloudy white film. Disturb that film, and it would grow back almost immediately. “I’d throw a pebble in the pool and knock the little scum of carbonate down, and a day later, a new one would have formed,” says Kelemen, who is the Arthur D. Storke Memorial Professor in the Department of Earth and Environmental Sciences. “For a geologist, that’s just superfast. That’s supersonic.”
So when he and fellow Columbia geochemist Juerg Matter returned to Oman on a field trip for students in 2007, they collected samples of the carbonate and had them dated using carbon-14 analysis. “I thought a lot of these deposits would be millions of years old, but they weren’t,” Kelemen says. “It turned out they were all less than 50,000 years old.” Their tender age was a strong indication that the carbonate deposits were still forming. This seemed to happen when the mountains’ topsoil eroded to expose fresh peridotite and when new cracks appeared in the rock, permitting carbonate deposits to form deep inside.
Kelemen and Matter estimated that the peridotite was absorbing about 100,000 tons of carbon annually, far more than geologists had previously believed. Kelemen and Matter soon published a study theorizing that this natural process could be accelerated a million-fold in some places. Their idea was to pierce the Hajar Mountains, a range the size of Massachusetts, with boreholes and then pump huge quantities of CO2-infused water into the ground. Even considering the economic and engineering challenges involved, Kelemen and Matter concluded that it would be feasible to store several billion tons of CO2 per year, or roughly 10 percent of all of the CO2 that humans are now producing. Alternatively, they proposed drilling boreholes off the coast, which would permit seawater to permeate the mantle rock beneath the ocean floor and deposit its CO2 there.
Finding a way to store carbon dioxide in a mineral form has always been the Holy Grail of sequestration research, says Greg Dipple, a professor of geology at the University of British Columbia. “It’s been recognized since the early 1990s as the optimal way to store carbon, but it hadn’t been feasible,” he says. “What Peter came up with is a novel way to do it on a large scale.”
Rock-Tight Storage?
This past January, Kelemen spent a month in Oman collecting more samples.
“We’re now interested in learning how to maximize the amount of CO2 that a certain volume of rock absorbs,” he says. “What nature can do in this regard is amazing — we’ve found pieces of the rock that literally have carbonate attached to every single one of the magnesium and calcium atoms. It’s permeated. Maxed out. And we want to learn how to make more of the rock like that.”
In recent years, Kelemen has learned more about the mechanism by which peridotite absorbs CO2. For example, he has discovered that when carbonate forms within the cracks of peridotite it presses out against the surrounding rock, forming new, microscopic fissures. These clefts allow more air and water inside the peridotite, which kick-starts a self-perpetuating cycle of carbonate formation and splintering.
“This rock from the earth’s interior is out of equilibrium with our atmosphere, and hungry for carbon dioxide,” Kelemen says. “We want to take advantage of that. This is chemical potential energy, as a geochemist would say. It’s there to be harnessed on a massive scale, if we can learn how to do it.”
Kelemen is now collaborating on the project with several other Columbia scientists. Among them is Heather Savage, a geophysicist who studies earthquakes. She is now applying everything she knows about how rock formations near tectonic faults naturally slip, crumble, and crack in order to help Kelemen figure out a way to initiate new fissures within peridotite, thereby exposing more of its surface area to CO2. Alissa Park, a climate scientist and engineer who has done extensive research on novel methods of carbon sequestration, is working with Kelemen to understand the optimal conditions for CO2 to chemically combine with elements in the peridotite.
“One trick is to circulate the water as deep into the ground as possible, because heat from the earth’s interior is going to make the chemical reactions occur at a faster rate,” says Kelemen.
Kelemen’s next goal is to dig a single borehole and experiment with the injection and removal of large quantities of CO2-rich water. The operation would cost roughly $10 million, and so far he has not convinced anybody to put up the money. Part of the challenge in funding his work, Kelemen says, is that private companies don’t yet see commercial potential in storing carbon dioxide, especially when scientists are still searching for an economically viable way to remove it from the air.
He is prepared to be patient. “Maybe in ten or twenty years, after we’ve had catastrophes that are clearly attributable to global warming, there will be more urgency surrounding the development of these technologies,” Kelemen says. “When the time comes, we want to have the basic concepts ready to go.”