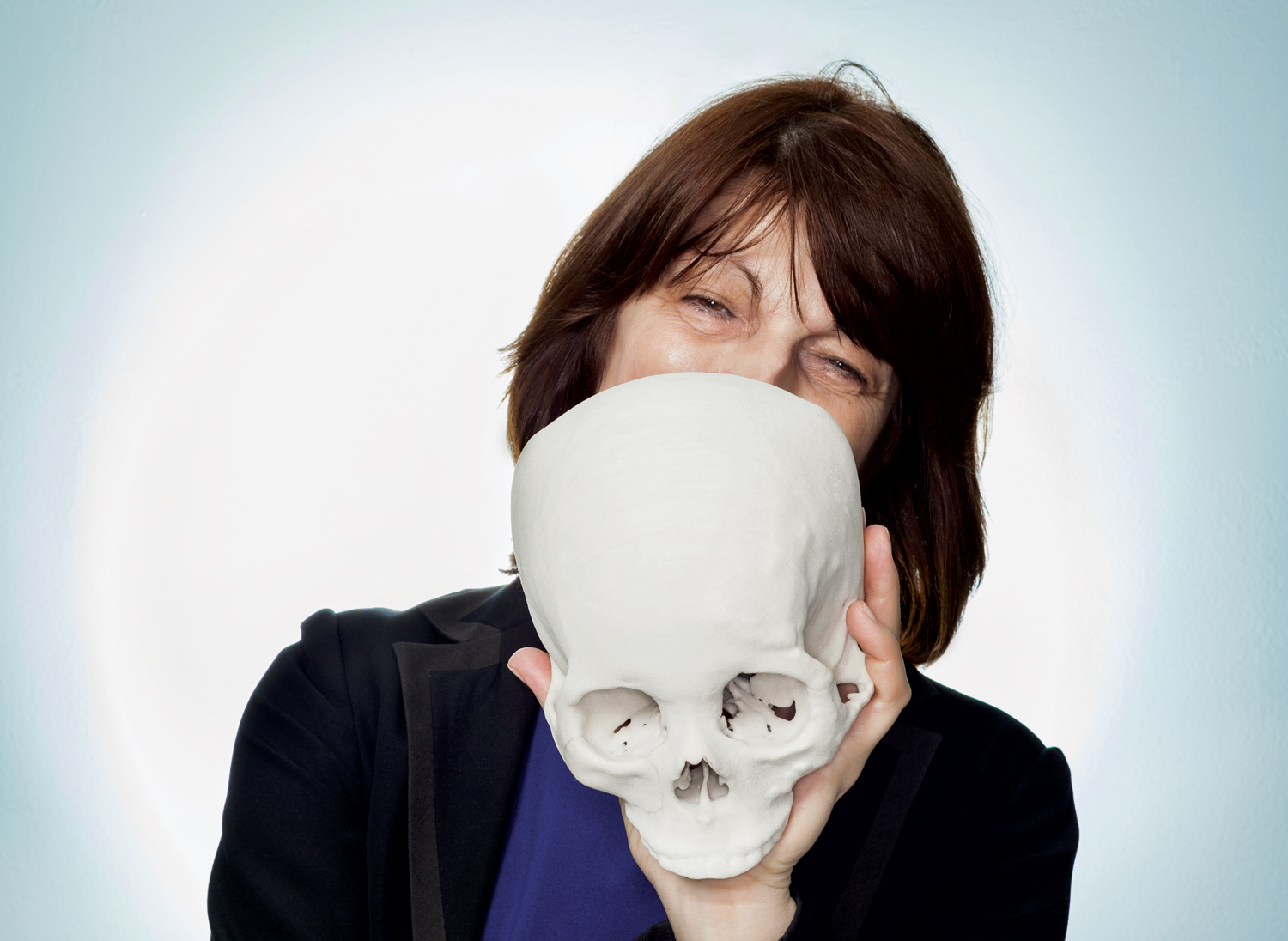
Jeffrey Ascherman had seen dozens of patients who looked like Marika, an eleven-year-old girl from Uniondale, New York. She had the drooping eyelids and sunken, formless cheeks of someone afflicted with Treachery Collins syndrome, a hereditary condition that causes people to be born without certain facial bones.
Her parents were hoping for a cosmetic fix. Marika, an honor-roll student, violinist, and aspiring actress whose name has been changed for this article, dreamed of looking more like her classmates.
But Ascherman, a plastic surgeon at the Columbia University Medical Center (CUMC) who specializes in treating children with cleft palate and other facial deformities, had no easy solution to offer. Implanting prosthetic bones was not an option for a still-growing child. The only way to fill out Marika’s face, Ascherman told her parents, would be to make an incision across the top of her head, remove a portion of her skull, and carve it into the shapes of two cheekbones. If Marika was lucky, the bones, once implanted, would grow along with the rest of her face.
But this solution was not ideal. The recovery would be long and painful as the skull bone grew back. Even then, there was no guarantee the procedure would work. Over time, the grafted bone might simply dissolve in her face, requiring reparative surgeries and more suffering.
Unpleasant as this was to contemplate, Ascherman knew that, to the girl and her parents, doing nothing seemed unthinkable.
“This is a condition that often leads to social withdrawal and ostracism,” he says. “When you see what these kids go through, it breaks your heart.”
Ascherman, who sees a handful of children with Treacher Collins every year, had resigned himself to the limited options available until he learned about the work of Gordana Vunjak-Novakovic, the Mikati Foundation Professor of Biomedical Engineering at Columbia’s Fu Foundation School of Engineering and Applied Science. Last July, he heard Vunjak-Novakovic deliver a lecture about her efforts to grow living human tissue. Afterward, he approached her and asked if she might grow facial bones for his young patients.
“She said anything was possible,” he recalls, “and that she was willing to try.”
Last fall, a team of Columbia clinicians led by Sidney Eisig, a pediatric oral and maxillofacial surgeon, took a big step toward achieving this goal. They implanted in pigs — whose facial anatomy is similar to that of humans — jawbones that Vunjak-Novakovic had grown in her laboratory. Soon, Ascherman hopes to try the procedure with cheekbones. If the experiments prove successful, human trials could begin as early as next year.
Growing human facial bones is just one of the seemingly insurmountable medical challenges that Vunjak-Novakovic is now close to solving. She is also growing strips of heart tissue that beat with life, small pieces of lung, and chunks of cartilage that she hopes doctors will one day put into people like spare parts into automobiles.
Like many engineers in the nascent field of regenerative medicine, Vunjak-Novakovic works with adult stem cells taken from bone marrow or fat deposits. These cells are powerful tools for medical researchers because they are highly versatile, able to differentiate as replacement cells throughout the body so that our organs, bones, and muscles can recover from normal wear and tear. Once the stem cells are extracted, Vunjak-Novakovic places them into a three-dimensional mold in the shape of the tissue she wants to create. But it takes more than the right-shaped mold to tell these cells what type of tissue to become.
“Stem cells take their cues from the nutrients they receive, the intensity of electrical impulses they feel, how much oxygen they get, and even how much movement they experience,” she says. “All these factors, in addition to the physical dimensions of their surroundings, indicate to the stem cells what part of the body they’re in. We need to create an artificial environment that mimics all of that.”
When this works, as it has in a few initial experiments, Vunjak-Novakovic says, she feels a sense of wonder, as if she’s glimpsing the mystery of life itself: a tiny cell, containing within its DNA a blueprint for the entire body, develops into the one type of tissue she needs.
Because reproducing the right conditions for growth is so difficult, only a handful of biomedical engineers have ever succeeded in growing tissues that could be put into people. And these have been relatively simple tissues, like skin and blood vessels — nothing as complex as bone, lung, or heart tissue.
“If we manage to create a human bone, we will have participated in something amazing,” she says. “A brand-new bone derived from a patient’s own body would function very smoothly, since it would be a perfect genetic fit. This will be a game changer in medicine.”
Between worlds
Vunjak-Novakovic can look up from her desk on the twelfth floor of CUMC’s Vanderbilt Clinic and gaze upon her birthplace, Belgrade. On the far wall hangs a large aerial shot of the city, with its closely clustered medieval buildings, bracketed by the Sava and Danube Rivers.
In the early 1980s, when Vunjak-Novakovic was working toward a PhD in chemical engineering at the University of Belgrade, the prospect of growing body parts never occurred to her. She was interested in the forces and motions created by the intermingling of gas bubbles and tiny solid particles in liquids. Her research involved mathematical modeling and experiments in enclosed reactors, and was applicable, most obviously, to industries that rely on fermentation, like food production and the manufacture of penicillin and other antibiotics.
“My interest in engineering was quite intellectual and abstract,” she says. “I’ve always loved solving complex puzzles. I didn’t think much about the applications.”
Over the next few years, though, as a young faculty member at the University of Belgrade, she became captivated by the chemical interactions that take place among molecules within living organisms. In 1986, she won a Fulbright fellowship to pursue this interest and chose to spend her year at the Massachusetts Institute of Technology. There, she caught the attention of a researcher named Robert Langer, who was trying to purify human blood for use in medical experiments. He was looking for someone to create new machines to remove toxins from blood.
The two began a collaboration that continued after Vunjak-Novakovic returned to Belgrade, and in the years that followed she made biannual trips back to Boston. During one such visit, in 1991, ethnic tensions in her homeland boiled over into war.
“It became clear that it would be good to leave Yugoslavia,” says Vunjak-Novakovic. “So I began spending more time in Boston.” Concerned colleagues at MIT, upon learning in 1993 that her visa was about to expire, lobbied successfully to get her a permanent position that allowed her to stay in the US with her husband and young son.
Around the same time, Vunjak-Novakovic’s career took another turn: Langer informed her that he had received a grant to do something called “tissue engineering,” and asked if she would like to join the project.
“Tissue what?” Vunjak-Novakovic asked him.
Life's complications
The term “tissue engineering” had been coined a couple of years earlier to describe what were the first serious attempts to create living tissue through artificial means.
For a young engineer interested in this challenge, Vunjak-Novakovic was in the right place. Langer was about to develop some of the field’s most important lab techniques. His main contribution would be to create three-dimensional molds, or scaffolds, that could be seeded with developing cells and then put safely into a person’s body. These lattice-like scaffolds are typically made out of natural molecules, such as collagen, or synthetic materials designed to be biodegradable. This approach is still used by most tissue engineers today.
“One of Gordana’s roles in my lab was to manage the flow of nutrients in and out of that mold,” Langer says. “That’s extremely complicated. You’ve got multiple fluids swishing about, and you need to control where, when, and in what quantities they’re touching the developing cells.”
When Vunjak-Novakovic opened her own laboratory at MIT in 1993, her first project was to try to create cartilage, the flexible connective tissue that cushions joints. Many of the first tissue engineers, including Langer, were trying to make cartilage because it is among the body’s simpler tissues and seemed like a good place to start. Government funding agencies and private companies, recognizing the huge market potential for helping people with arthritis and other joint problems, invested heavily in these projects.
“The thing about cartilage is that it doesn’t connect to the body’s vascular system,” says Vunjak-Novakovic. “So from a technical standpoint, it seemed like the easy target.”
At the time, tissue engineers saw their primary challenge as determining the right mix of nutrients, minerals, and proteins to feed their growing cells. Even slight variations in the nutrient soup they injected into their molds had a profound effect. A little extra calcium, for instance, would signal to the stem cells to develop into bone.
“I think everybody assumed that was 99 percent of the game,” says Vunjak-Novakovic. “Could other factors be influencing these cells? Sure, perhaps. But the consensus was that if you had a well-shaped mold and the right nutrients, you were probably good.”
Vunjak-Novakovic, however, was reading a lot about systems biology at the time. She was fascinated to discover that many physiological systems — genetic, molecular, electrical, and mechanical — are interconnected in surprising ways. In particular, she noted that people immobilized in hospital beds for long periods of time often experience a weakening of their bones and cartilage. It seemed that physical movement was essential to the upkeep of these tissues. Was it possible, she wondered, that developing cells are also sensitive to movement?
This was an idea that many physicians and biologists had considered but never had the means to test. To do so, Vunjak-Novakovic conducted an experiment in which a piece of developing cartilage was periodically rotated upside down. After a few weeks, she tested its strength. She’d hit the scientific jackpot. “The improvement in its structural integrity,” she says, “was beyond what we’d imagined.”
This led to a technical innovation: Vunjak-Novakovic developed a plunger-like device that gently presses down on the chemical solution in which developing tissues are immersed, replicating the forces of movement experienced by stem cells in the body’s joints.
But the real advance was philosophical, Langer explains: “Since then, all tissue engineers have come to appreciate the need to consider lots of variables, besides the molecular factors. She helped inspire this shift in outlook.”
For her design of highly sophisticated bioreactors, or tissue molds, Vunjak-Novakovic was recently elected to the National Academy of Engineering, one of the profession’s highest honors.
Open house
Vunjak-Novakovic, a gentle-natured and cheerful woman who speaks with a soft Slavic accent, came to Columbia in 2005 intent on moving her technology from lab bench to bedside.
She knew she was going to need help. By this time, all biomedical engineers had come to realize that creating tissues capable of functioning in the human body would be much more difficult than they’d initially anticipated. “Even the cartilage wasn’t working as we’d hoped,” says Vunjak-Novakovic, who today also is a professor of medicine at the College of Physicians and Surgeons. “One of the odd things about cartilage, which makes it surprisingly difficult to work with, is that it has no natural ability to repair itself. When your cartilage deteriorates, that’s it — it doesn’t regroup. We discovered that stem cells, once they begin to identify themselves as cartilage, will get lazy and stop growing, too.
“Why nature would create a tissue with no ability to repair itself is one of life’s mysteries,” she continues. “The same thing is true of brain, heart, and pancreatic tissue.”
The body parts that biomedical engineers were making progress on were those that, in addition to having some capacity for self-repair, have large surface areas relative to their mass. This was because a thin piece of tissue, once implanted, would be well exposed to the body’s veins and capillaries, increasing the likelihood that it would receive the blood and oxygen it needed. Skin was in this category, as were some tubular structures such as the urethra, trachea, and intestines, as well as the sack-like bladder, which is among the body’s simplest organs. (Today, biomedical engineers have succeeded in growing and implanting in people all of these tissue types, in addition to blood vessels.)
At Columbia, Vunjak-Novakovic continued working on cartilage but shifted her focus to one of the most daunting targets of all: the heart. The fibrous muscle tissues that compose the heart have little capacity for self-repair. Also, it would be difficult to get engineered heart tissue enough blood. “The walls of the heart are quite beefy, nearly a centimeter thick,” says Vunjak-Novakovic. “So any heart tissue we created would need to have its own vascular infrastructure ready to work efficiently as soon as it went into the body.”
But Vunjak-Novakovic decided that it would be worth the effort to create a tissue that could save lives. And she figured that by exploiting the knowledge of Columbia’s physicians, cell biologists, and other engineers, she might at least make some progress. “I decided that a key strength of our laboratory,” she says, “was going to be our openness to collaboration.”
Among the first Columbia physicians who showed interest in her work was the cardiologist Warren Sherman. In 2001, he was one of the first clinicians ever to inject stem cells into a person’s heart as a way of facilitating the organ’s recovery. He did this a few days after his patient had suffered a heart attack, aiming the stem cells straight into the region of the heart that had been deprived of blood and oxygen. His goal was to help the damaged muscle grow back. A large-scale clinical trial later showed that the treatment was beneficial, reducing the chances of subsequent cardiac episodes.
This procedure had shortcomings, however. “The stem cells you shoot in there don’t have good survival rates,” Sherman says. “If you inject two hundred million cells, you might get 5 percent of them to start growing as muscle.”
Sherman hoped that Vunjak-Novakovic might create new tissue in physically intact strips that could be applied, like living Band-Aids, to the damaged parts of the heart. He’d heard that a few years before showing up at Columbia, Vunjak-Novakovic had discovered that stem cells are more likely to turn into heart cells if they get zapped with electricity in the laboratory.
“I’ll never forget when I stared down into the mold and saw those cells begin to twitch with life,” Vunjak-Novakovic says. “I thought the table was shaking at first.”
The link between electricity and heart cells didn’t surprise many biologists, since the heart starts to beat in a fetus only when it receives an electrical signal. But it was another example of a crucial variable that other tissue engineers had not yet tested or incorporated into their laboratory techniques.
Today, Vunjak-Novakovic is working on a heart study with a team of Columbia faculty that includes Sherman; his fellow cardiologists Robert Kass, Shunichi Homma, and Lynne Johnson; biomedical engineer Elisa Konofagou; and stem-cell biologist Christopher Henderson. With grants from the National Institutes of Health and the private Helmsley Charitable Trust, they are cultivating heart cells in a bioreactor that Vunjak-Novakovic has configured to mimic the physical environment of a heart chamber. With her colleagues’ guidance, Vunjak-Novakovic has replicated the heart chamber’s temperature, moisture level, electrical stimulus, and even the gentle flushing motions of blood flow.
“We each benefit from this arrangement,” says Kass, the Alumni and David Hosack Professor of Pharmacology and the chairman of that department. “Scientists who study the electrical properties of heart cells, as I do, have traditionally been limited to observing isolated cells. In Gordana’s laboratory, we’re now able to see how groups of heart cells interact with one another in a living system.”
Hearts and bones, locally grown
Vunjak-Novakovic now believes she is on the cusp of creating a heart patch and facial bones.
She recently tested a patch in mice that helped repair the rodents’ hearts after they had been damaged by mild, localized heart attacks.
“This was one of the first heart patches to provide much benefit to its recipient, even in small animals,” says Vunjak-Novakovic. “What’s often happened, until now, is that the new tissue wouldn’t beat very enthusiastically along with the rest of the heart.”
Challenges still must be overcome before Vunjak-Novakovic can create a heart patch for humans, though. Chief among them is properly connecting the new tissue’s tiny blood vessels to the organ’s existing vascular system. This plumbing problem, she says, remains a frustration to all biomedical engineers working on complex tissues.
“The blood vessels in the lab-grown tissue always seem to close in on themselves, shutting themselves off from the outside,” says Vunjak-Novakovic, who is currently seeking grant support for a heart-patch study on pigs. “That’s why our results with the mice are exciting. It’s going to be even more challenging to make it work in larger animals, but we’ve got a good shot.”
Meanwhile, Vunjak-Novakovic thinks she is close to revolutionizing the treatment of some craniofacial problems. Recently, she has been developing a method for growing jawbones and cheekbones to help people who have suffered traumatic injuries or been born with congenital deformities, like many of the children that Jeffrey Ascherman sees. Children could benefit the most from her work in this area, Vunjak-Novakovic says, since full-grown adults typically have the option of receiving plastic prosthetic bones. But even adults may benefit from lab-grown bones, because prosthetics can cause side effects, like inflammation, and they need to be replaced after ten or fifteen years.
“We’re now able to grow a full-size jawbone or cheekbone within three weeks,” she says. “And doctors say the wait would be worth it, in many cases.”
Bones, too, are very difficult to engineer. In addition to the problem of connecting them to the body’s vascular system — all bones have at least one or two blood vessels — there is the additional complication of convincing the body’s ligaments, tendons, cartilage, and muscle to hold them in place. Vunjak-Novakovic thinks she’s found a solution. She is now about halfway through the study in which Sidney Eisig, the oral surgeon, has given pigs jawbones that Vunjak-Novakovic created using their own stem cells. Preliminary results suggest that the bones are binding properly to the native connective tissues. Vunjak-Novakovic won’t disclose exactly how she accomplished this, but she says it involves the precise timing of the bone’s implant.
“Basically, you cultivate the bone in your lab until it’s ready to function in the body, but not so long that it starts to think of itself as an independent system,” she says. “You put it in the body the moment nature is ready.”
Learning to work with nature is, to Vunjak-Novakovic, the essence of regenerative medicine. She regards herself not as a creator of tissue but as a sort of lab-coated shepherd guiding the body’s own powers of growth and healing.
“The only real tissue engineers are the cells in our body,” she says. “The people on my team are toolmakers, builders. We make little physical environments that help cells do what they’re capable of doing. If your bone breaks, it wants to grow back. But maybe it’s gotten old and cannot recover like it used to. We’re tricking stem cells only in the sense that we’re putting them in an environment where they can feel how young and strong the body is capable of being again. I believe that.”