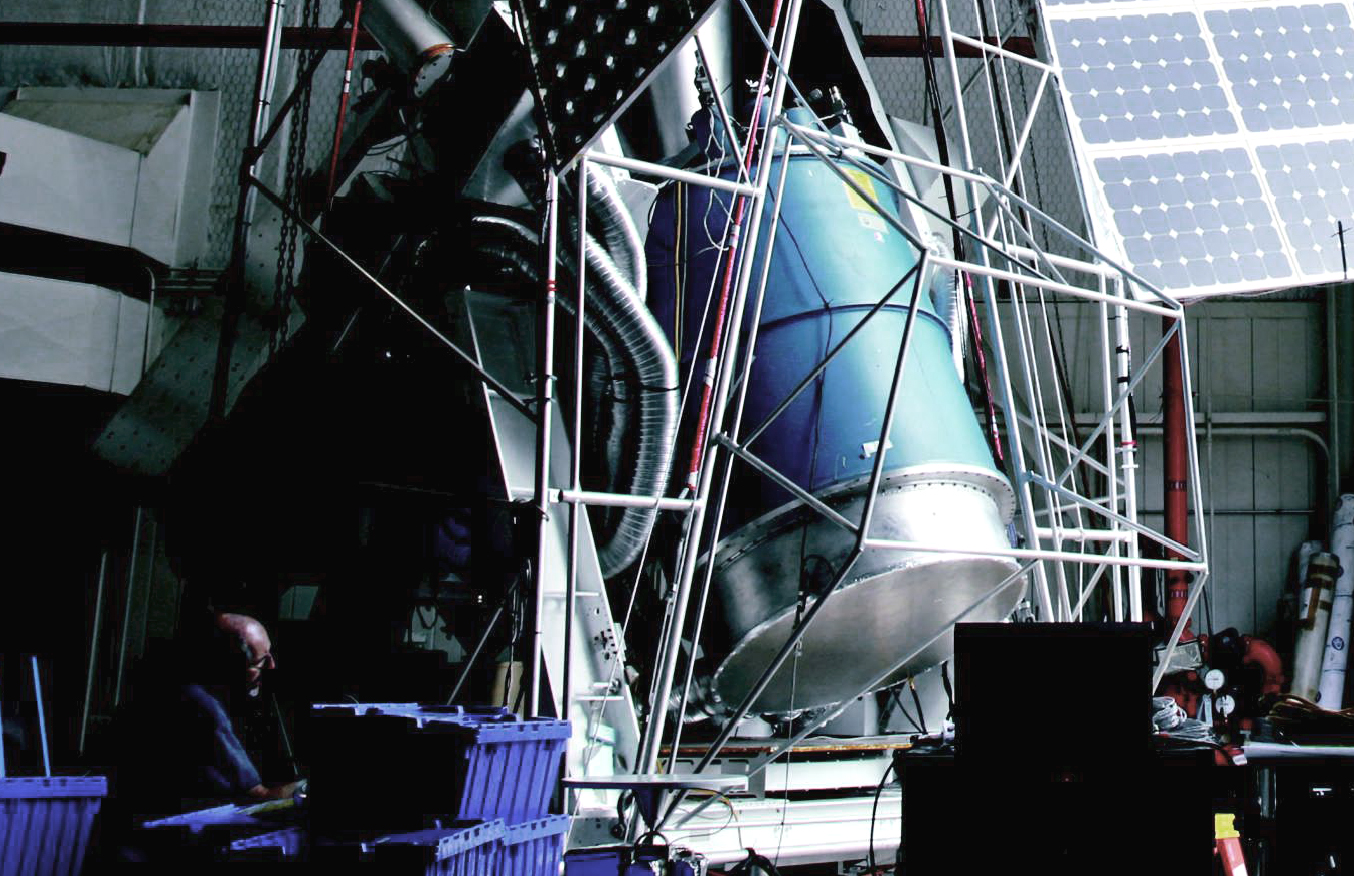
It was the night of December 13 when the C-130 military transport carrying Amber Miller and a group of other researchers entered the frigid airspace over Antarctica. Miller had been traveling for nearly ninety-six hours. Still, she kept her eyes glued to the window, where a serene light was waiting to greet her. The approaching tundra was suffused in the eerie glow of the midnight sun.
“It’s spectacularly beautiful,” she says. “You can see the glaciers and the snow flowing like a river down these big mountains, and you can see icebergs and ice floes, and everything is white, white, white.”
Miller had come to Antarctica looking for light, but not the kind one sees from a plane. The light she’d come looking for was about 13.3 billion years old, and potentially more revealing, at least to cosmologists, than any other light in the universe. This light was created milliseconds after the Big Bang, and for the past seven years, Miller, an experimental physicist and the dean of science for Columbia’s Faculty of Arts and Sciences, had been leading the effort at Columbia to build a telescope that could detect it.
“We’re talking about a light from so far away that it has taken the entire history of the universe, from before galaxies ever formed, to get here,” she says. “We’re seeing the edge of the observable universe.”
As head of the Columbia team working on the E and B Experiment, better known as EBEX, Miller was one of the prime architects behind a 6,000-pound, balloon-borne telescope. Depending on what that telescope sees, it could either prove or disprove a key group of ideas about the universe and its origins.
“We want to actually observe what happened in the first 10-35 seconds after the Big Bang,” she says. “Just that instant of creation.”
The ideas in question are known collectively as inflation theory, and they address several longstanding mysteries. For example, when viewed over a large distance, why does the universe appear flatter than it should? And why does the universe seem to be much larger than it should be, based on the rate that it’s currently expanding?
The answer, according to the most basic understanding of inflation theory, is that the universe hasn’t always expanded as slowly as it does now. Instead, for the briefest of moments following the Big Bang, the universe actually expanded faster than the speed of light.
If inflation theory is accurate, then that rapid expansion would have left a unique but faint signature on the cosmos. “The universe would have undergone a period of superluminal expansion during which space-time would have been stretched and compressed,” Miller says. “That produces gravitational waves, and those waves then travel through space-time.”
At the time inflation supposedly took place, the brand-new universe was too dense and hot for any light to escape. And it stayed that way for about 380,000 years. But the gravitational waves created by the rapid expansion would have left an impression on light that was emitted when things began to cool down. That light, cosmic microwave background radiation (CMB), is known to exist because scientists have already detected and photographed it, although not with instruments sensitive enough to detect the imprints of those gravitational waves.
EBEX is equipped with detectors capable of sensing the faintest hint of these impressions.
“If you can detect this very special signature,” says Miller, “you’re actually looking at information that comes from the instant that the universe was created.”
Much like a twenty-megapixel camera will produce detail on a photo that a ten-megapixel camera never could, the EBEX telescope represents a technological leap forward, an instrument sensitive enough to read eons-old fluctuations that were previously undetectable.
“We’re looking for a small signal in a big background,” says Britt Reichborn-Kjennerud ’10GSAS, a postdoctoral fellow who worked closely with Miller on the project.
While the scientists could have done this work elsewhere — CMB exists in every part of the universe — Antarctica proved to be the friendliest environment, in part because the Antarctic winds blow in a circle, so the telescope could finish its journey more or less where it began. “You need your experiment to come back to a recoverable place,” says Miller. “You can also get a long flight without having to fly over anything you’re not allowed to fly over.”
Building the telescope required the collaboration of seventeen institutions, chief among them the University of Minnesota, which built the camera that took the pictures, and NASA, which funded the project and managed the launch. For seven years, Miller’s team constructed, tested, and adjusted the telescope, as well as the camera and the gondola to carry it. Late last year, the entire instrument and fifteen members of the crew made the journey to Antarctica. For some members of the team, Antarctica became home for more than four months.
On December 28, the flight crew prepared for the launch. The temperature had dropped to minus nine degrees. For nine hours the crew waited on the ice for just the right conditions. Finally, the balloon billowed upward, casting a shadow that grew and swept rapidly across the ice, lifting the EBEX telescope high into the atmosphere. “It was timed so perfectly that EBEX seemingly just gracefully slid off the pin and floated upward,” wrote a member of the crew on his blog.
With a seven-month-old infant at home, Miller had to leave Antarctica a week before launch. “I was watching it over the Internet and on the phone and on the computer,” she says. “It was heart-wrenching.”
For two weeks, the polar-vortex winds carried the telescope in a circle above the South Pole as it collected data. It landed on January 24, completing a nearly month-long journey around the pole.
Fully analyzing the data will take years, and it will be at least several months before scientists will know whether they found the elusive signals they sought.
“If the signals are there,” says Miller, “it’s a smoking gun for inflation, because there’s no other reason they should be there.”
If the signals aren’t there, that will be valuable, too. “Different inflationary models predict different amplitudes of signal,” she says. “So not finding the specific signal we’ve been looking for would at least narrow down the possibilities of how the universe began.”