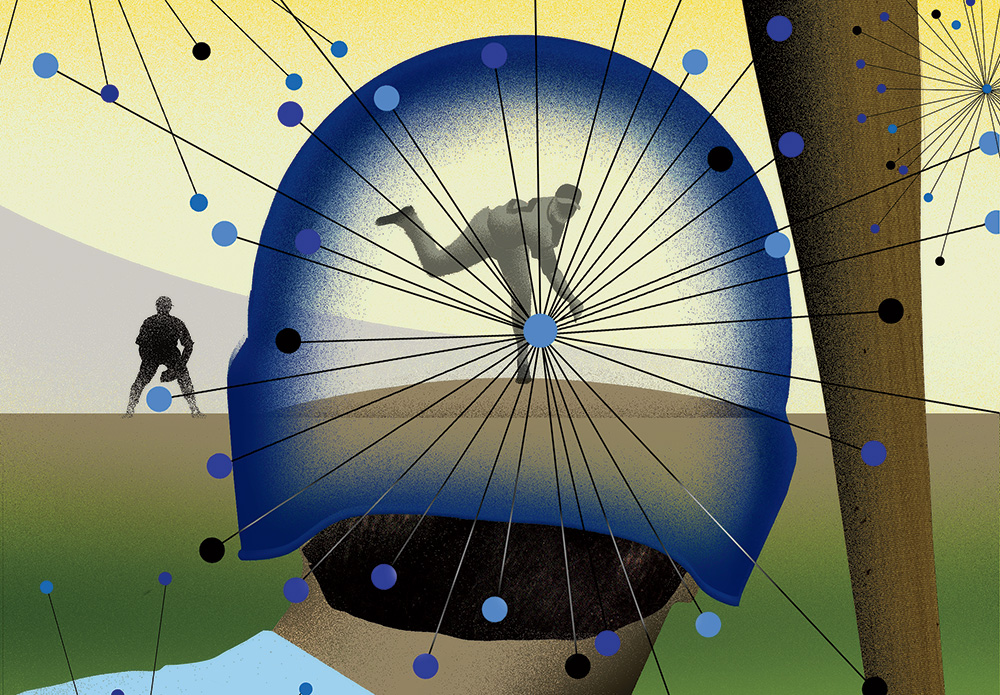
Gene Larkin ’84CC was shaking. “I’ve never been so nervous in my life,” he says.
It was October 27, 1991, and the Minnesota Twins and the Atlanta Braves were tied 0–0 in the bottom of the tenth inning of the seventh game of what many consider the greatest World Series ever played. With fifty-five thousand fans rocking the Metrodome in Minneapolis and another fifty million watching on TV, the Twins loaded the bases with one out. If they could bring the runner home from third base, victory would be theirs. But the next man up was known for his speed, not his bat, and Twins manager Tom Kelly decided to make a switch: with the series on the line, he went over to Larkin, a twenty-nine-year-old bench player with knee tendonitis, and said, “It’s your turn.”
Larkin had been summoned to pinch-hit six times in the postseason and had reached base once. Knowing he might be called again, he’d started doing stretches and swinging a bat in the fifth inning, though in fact he’d been preparing ever since he was a boy in North Bellmore, on Long Island. Back then, like any kid playing ball in his yard, he’d pretend he was at bat in Game 7 of the World Series with a chance to win the whole thing. Now, somehow, the fantasy had materialized in all its surreal intensity, and as Larkin lumbered from the dugout into the thousand-watt lights, he was shaking so badly that he was sure everyone could see it.
But when he stepped into the batter’s box and looked out at the pitcher, Alejandro Peña, something strange happened: Larkin entered a tunnel of calm. “It was just so weird,” he says. “I went from the most nervous person ever to ‘I’ve been in this batter’s box thousands of times.’” The Braves outfielders were playing shallow to cut off the winning run, and Larkin was looking for a first-pitch fastball to send over their heads.
For most mortals, making any contact with the ball would have been next to impossible. A pitch traveling at ninety miles per hour from the pitcher’s hand to the catcher’s glove takes about four-tenths of a second, and it takes a full two-tenths of a second for the brain to send a signal to the muscles to respond. “That leaves your brain with two-tenths of a second — half an eye blink — to be able to see the pitch as it’s coming in and decide whether or not to swing,” says Zach Schonbrun ’11JRN, author of The Performance Cortex: How Neuroscience Is Redefining Athletic Genius. The decision is complicated by other factors: the type of pitch (fastball, curve, slider), its location (high, low, inside, outside), the situational stress (in Larkin’s case, extreme), the noise of the crowd (ditto). “Anything that clouds the hitter’s focus eliminates the possibility of squarely hitting the ball,” Schonbrun says. “There’s just not enough time during a pitch for a hitter to readjust.”
As Peña stared down at his catcher for the sign, Larkin cocked his bat repeatedly. His task was to bring the thickest part of the bat, called the barrel — about two and a half inches in diameter — into precise contact with a speeding 2.9-inch-diameter projectile.
Peña kicked and delivered, and as the ball left his hand, Larkin reacted: his retina transmitted information to his visual cortex, which signaled his motor cortex, which sent nerve impulses down his spinal cord to his muscles. His arms extended, the barrel met the ball, and in that instant of contact, Larkin knew.
The fans knew too, and so did the CBS television announcer, Jack Buck, who at the crack of the bat said, “The Twins are going to win the World Series!” — and then, as the arcing ball landed on the turf beyond the outfielders: “The Twins have won it! It’s a base hit! It’s a 1–0, ten-inning victory!”
The Metrodome nearly burst its roof as Larkin, arm raised, loped to first base while the man on third scored the winning run, and Larkin’s teammates rushed onto the field to mob him. “I went from nervous as hell to calm to exhilarated in ten seconds,” he says.
For decades, Columbia psychologists, biomedical engineers, and therapists have been probing the secrets of athletic excellence, the integration of mind and body that allowed Larkin to succeed. And today, at Columbia’s Zuckerman Institute, neuroscientists are revealing the mechanics of movement itself, from basic voluntary actions to tasks requiring quick decisions, elaborate coordination, and memory. This research into the orchestration of neurons and muscles, the poetry of motion, could not only help us improve our performance at work and at play but also lead to treatments for motor impairments caused by injuries, stroke, and diseases such as Parkinson’s. Unlocking athletic success, and controlled movement generally, is both a brain game and a mind game, a cerebral double-header, and it all begins with that three-pound ball of nerve cells in our skulls.
“To understand the brain is to understand action,” says Daniel Wolpert, a neuroscientist at the Zuckerman Institute and a leading authority on motor control. “We tend to think our brain is there for us to perceive the world, but the brain’s most basic function is to move our bodies, whether to evade a threat or to interact with others through speech or gestures. The only way to affect the world is through the control of movement.”
For an athlete trying to hit a ball or shoot a basket, action is complicated by the potential range of our movements: “It’s hard to control things precisely,” Wolpert says. “With two hundred joints and six hundred muscles, we have many degrees of freedom. Every time we try to use our muscles there’s variability.” Wolpert calls this variability “noise” — the inherent inconsistency that prevents us from generating the same exact motion each time we swing a bat or chop a carrot. “Motor noise increases the faster you move. If you do something very slowly, you can do it precisely. If you try to do something quickly — like swing a bat — it adds more variability to the movement.”
Another problem is that the electrical connections in the human nervous system are far slower than those of smaller animals. Whether we’re biking in traffic or trying to hit a moving ball, there is a delay between the eye seeing and the muscles reacting. “It takes time for the retina to respond, for the information to get to your brain, and for the body to respond,” says Wolpert. “That means that when you want to make fast movements in sports, you have to predict ahead of time. Prediction is fundamental. You have to anticipate where the ball will be.”
The element of prediction is as complex and subtle as it is central, explains Nathaniel Sawtell, an associate professor of neuroscience at the Zuckerman Institute who studies how the brain filters out unimportant information so that we can focus on a task. “Any time you’re moving around in the world, you’re bombarded by sensory input that’s a result of your own movements, and you need to be able to tell whether a sensory input is due to an external event or your own actions,” Sawtell says. “And so the brain has to constantly generate predictions of what sensory input to expect from a given movement. If you pick up an object that looks light but is really heavy — say, a cup you thought was empty but is in fact full — your arm will jerk up, which shows that you made a prediction about the cup’s weight.
“The simplest actions involve complicated coordination, and you want to use these predictions. Still, every so often, you screw it up.”
Larkin, plucked from the bench with the bases loaded in the tenth inning of the deciding game of the World Series, faced these challenges and more. “You can take all the batting practice before a game, do all the mental exercises, and study all the film on the pitchers,” says Larkin, who retired as a Twin in 1994 and today lives in Eden Prairie, Minnesota, where he works as a financial planner. “But when you’re in the batter’s box and there’s a guy sixty feet and six inches away throwing ninety-five miles per hour, you are on an island, and no one can help you.”
So what, exactly, was happening in Larkin’s brain as the ball left Peña’s fingertips?
That’s what Jordan Muraskin ’15SEAS wanted to know. As a PhD student at Columbia, Muraskin worked in the lab of Paul Sajda, a professor of biomedical engineering who focuses on what occurs in our brains when we make split-second decisions. The Sajda lab uses EEG (electroencephalography, in which electrodes attached to the scalp record electrical activity in the brain) and fMRI (functional magnetic-resonance imaging, which measures cerebral blood flow) to create temporal and spatial maps of the brain in action. In 2013, Muraskin, a Yankees fan, and postdoctoral researcher Jason Sherwin, a Cubs fan, employed this technology to launch a landmark study of the brains of athletes.
The cumbersome medical equipment precluded any trials with live pitching. Instead, Muraskin and Sherwin used physics equations to create computer simulations of the trajectories of three types of pitches: fastballs, curveballs, and sliders. Their test subjects, wearing video goggles and EEG caps and placed inside an fMRI scanner, saw a gray screen with a green ball coming at them in the motion of one of the three pitches. The fMRI would show where things were happening in the brain as the pitches came in, and the EEG would show when. Muraskin and Sherwin detected variations in the way the brain noticed different pitches. Excited, they took the idea to Sajda, a Mets fan.
Sajda loved it, and Muraskin had his doctoral thesis: he would combine the data from the EEG and the fMRI to illuminate what happens in the brain when people make hair-trigger decisions. He was particularly interested in comparing the brain activity of athletes to that of novices, and so he recruited members of the Ivy League champion Columbia baseball team (the Lions won Ivy titles in 2013, 2014, and 2015) to put on the goggles and “play ball” while the machines recorded the fluctuations of blood and electricity in their brains.
The results were eye-opening. In one finding, the investigators discovered activity in the fusiform gyrus, in a region called the fusiform face area (FFA), a part of the brain first named in 1997. “The theory was that faces are so important to us that there’s a part of the brain specially dedicated to them,” says Sajda. Later studies showed that the FFA also lights up in birdwatchers when they glimpse a bird. “The discussion became: maybe this area responds not just to faces but also to other objects that are important to us, and we file them there because there are neural connections between this area and the motor cortex that allow us to respond quickly. In the ballplayers, the pitches activated the FFA, which is really interesting: a pitch is not an object, it’s a ball moving in time. Yet it’s so important to the player that the brain learns to represent it in these privileged areas.”
In another experiment, the subjects were told to expect fastballs and to swing at them. If a curveball came, they were to not swing. This test activated a part of the brain called the pre-supplementary motor area, which is typically involved in inhibiting response. “When hitters are primed for that fastball, they’re a loaded spring,” Muraskin says. “Stopping that swing is as forceful a physical action as following through.”
According to Mark Churchland, an assistant professor of neuroscience at the Zuckerman Institute and an expert on voluntary movement, two things happen in the motor cortex before our bodies make a voluntary move. “The first stage is purely preparatory, and while it doesn’t commit you to ultimately making the movement, it’s necessary for movement, and that takes at least fifty milliseconds,” Churchland says. “Then there is the triggering stage, when you’re committed to the movement you’re going to make. As a hitter you might be able to struggle and check your swing, but basically, once you’ve pulled the trigger, you’re going to make that movement. You can kind of see athletes struggling with whether to pull the trigger. There can be a real cost to making a movement you didn’t want to make.”
With that truth in mind, Muraskin and Sherwin adapted their research on athletes and created deCervo, a company that develops simulation apps to help athletes, referees, and law-enforcement officers improve their decision-making. A deCervo video course for police officers incorporates real bodycam footage and provides feedback on the speed and aptness of users’ actions. The principles apply across fields, and one of the most compelling findings from the Sajda lab research was that the motor areas that govern inhibition were far more active in the ballplayers than in the non-players.
“What it looks like mechanistically is that what makes some hitters better than others isn’t how fast they swing but how fast they can stop their swing,” says Sajda.
In baseball this is known as having “a good eye” — the ability to judge the path of a pitch as soon as it’s released, and, if it’s out of the strike zone, to not pull the trigger. But such radical motor control isn’t the only tough demand on a batter. Early on, a player must overcome the primal fear of getting drilled by a whistling baseball. “This game is a crazy pursuit, because when you’re batting you’re always inches from getting hit,” says Jordan Serena ’15CC, a Columbia baseball star who played for three years in the Los Angeles Angels farm system and now runs the hitting program at Rogue Baseball Performance, a sports-training facility in Colorado. “Your brain sees a ball coming at your face, and it wants to save you. It wants you to move. But you’re playing a sport where you have to stand there, without freezing up or flinching, in case the ball comes down into the strike zone. Hitters at all levels freeze at the plate on certain pitches.”
The reflex of “freezing” in response to a sensory threat signal — a loud bang, the sight of a predator — has been observed throughout the animal kingdom. Richard Mann, a professor of biochemistry at the Zuckerman Institute who studies the neural circuitry that governs coordinated movement in fruit flies, runs a lab that recently uncovered the chemical source of this “startle response”: serotonin, a neurotransmitter known for its role in regulating mood. “Serotonin can change the physiology of the neurons that it acts on,” says Mann. “We think it gets released in the ventral nerve cord of the fruit fly, which is the equivalent of the spinal cord in vertebrates. We’re trying to identify the primary neurons that serotonin has to work on to cause the fly to freeze.” Mann posits that a good hitter must be able, whether innately or through training, to suppress the startle response.
The fruit fly has been informing our own molecular makeup ever since evolutionary biologist Thomas Hunt Morgan established his famous “fly room” at Columbia in 1910 and demonstrated the physical basis of heredity, revolutionizing the field of genetics. In the Mann lab, students and postdocs study the growth and function of the six legs of the fly, tracing the development of motor neurons from stem cells and trying to understand the circuitry that makes a fly walk. “When our limbs make a move, we have to coordinate all the different joints, flexors, and extensor muscles, then coordinate between the left and right legs, then the different segments,” says Mann. “Flies also do this — they use their legs to walk, groom, fight, and mate, and they have to coordinate all the movements. There are about fifty motor neurons for the leg and about fourteen muscles, so it’s not that complex a system. But it’s all translatable to vertebrate circuitry.
“Coordinating the muscles is where the rubber meets the road. No matter how complex your brain is, the execution is what matters, and in baseball, like any activity, every step of the process is important: how well you see, how well you calculate the speed and trajectory of something coming at you and then integrate that and respond to that within milliseconds. It’s astounding, right?”
On a September afternoon in 1921, the most famous man in America came to Columbia to take an exam. At twenty-six, George Herman “Babe” Ruth, outfielder for the New York Yankees, was a scientific curiosity. That year, Ruth would compile perhaps the best hitting season of any player in history: he would swat fifty-nine home runs (topping his own record of fifty-four set in 1920, which had smashed his record of twenty-nine set in 1919), drive in 171 runs, score 177 (still the most by any player since 1894), amass 457 total bases (an all-time record), and bat .378. Everyone wanted to know: how did he do it?
One day, after a game at the Polo Grounds (the Yankees’ home field) in which he’d hit one of his fabled long home runs, Ruth, still in his baggy gray uniform, accompanied the sportswriter Hugh Fullerton, on assignment for Popular Science Monthly, to Morningside Heights. The two men stopped at the Columbia campus and entered Schermerhorn Hall, home to the school’s psychological research laboratory.
Ruth’s visit stemmed from a burgeoning effort within psychology to analyze motor responses to predict success in athletics and other fields. Columbia was one of the centers of the young science. In 1891, James McKeen Cattell, whose early work had focused on how quickly a person could identify letters, words, and colors, was named head of Columbia’s new psychology department. Among his students was Robert S. Woodworth 1899GSAS, whose analysis of motor behavior and skilled performance led to his groundbreaking dissertation, “The Accuracy of Voluntary Movement.” And in 1915, the department hired E. W. Scripture, a pioneer in reaction-time experiments on athletes.
In Schermerhorn, two researchers, Albert Johanson 1916CC, 1922GSAS and Joseph Holmes, greeted Ruth and led him through a battery of standard psychological tests. For three hours, the Babe was the picture of steadiness as he performed the tasks. He jabbed a tiny peg into tiny holes as quickly and accurately as he could, tapped a key with his finger as fast as he could, pushed buttons in response to flashes of light, looked into a machine in which groups of letters of the alphabet were exposed for one fifty-thousandth of a second and called out the letters the instant he saw them. As Fullerton would write, Johanson and Holmes “figuratively took [Ruth] apart, watched the wheels go round; analyzed his brain, his eye, his ear, his muscles; studied how these worked together; reassembled him, and announced the exact reasons for his supremacy as a batter.”
The article was published in the October issue, which featured Ruth on the cover. “The secret of Babe Ruth’s ability to hit is clearly revealed in these tests,” Fullerton proclaimed. “His eye, his ear, his brain, his nerves all function more rapidly than do those of the average person. Further, the coordination between eye, ear, brain, and muscle is much nearer perfection than that of the normal healthy man.” The New York Times picked up the story, touting the conclusions in a breathless headline: ruth supernormal, so he hits homers / psychologists prove co-ordination of eye, brain, nerves and muscle is virtually perfect.
A hundred years later, that verdict appears as bloated as a mid-career Babe after an all-nighter at the Cotton Club. “There’s a narrow range of reaction times that all people fall into, whether it’s you, me, or Babe Ruth,” says Performance Cortex author Schonbrun. “I think if they’d brought in the backup second baseman of the Detroit Tigers, they’d have found similar results.” Richard Abrams ’79SEAS, a cognitive psychologist at Washington University in St. Louis who in 2006 put Cardinals slugger Albert Pujols through similar tests, agrees. “It’s not clear how the results could be used to predict success in sports,” he says. “While people who perform poorly are not likely to become good athletes, my guess is that there are people who perform really well who also won’t become baseball players.”
So what really separates the great players from the good ones? Daniel Wolpert, the motor-control expert, believes that part of the answer lies in nature. “I expect that great players have less variability in their motions and better learning algorithms than people like me, and are probably born that way,” he says. “I’ve tried to learn sports, and however much I try, I can’t improve in the way that others do.”
For Larkin, who was the first Columbian to make the majors since Lou Gehrig, there’s an X factor in performance that can’t be easily quantified using electrodes or vision tests. “You have to have an inner bravado at home plate and really believe that you can compete with the guy on the mound, no matter how good he is,” he says. “If you don’t, your chance of success is almost zero.”
But how to get there? Mark Louie ’19TC, a licensed professional counselor and assistant athletics director for championship performance at Columbia Athletics, emphasizes self-awareness: having a clear goal and knowing what you need to succeed. “If you have self-awareness and you know what motivates you and what your strengths and weaknesses are — if you know you’re going to perform better with certain resources available — then you can control some of those variables and give yourself the best opportunity to perform at a high level,” says Louie, who works with Columbia’s coaches and over 750 student-athletes.
Louie says that the best guarantor of success — whether it’s hitting balls, arguing a court case, or playing music — is mastering the skills that allow for optimal performance. “Mastery comes from deliberate and specific practice and is connected with increased resilience and confidence, which can prevent you from internally defeating yourself,” he says. “Confidence is a huge component of any performance.”
“Confidence is built by being very selective in how you manage your memories (not dwelling on past failures), how you talk to yourself in the present, and how you think about your future,” says Nate Zinsser ’82TC, director of the performance-psychology program at West Point and author of The Confident Mind. “In high-pressure situations you can’t become preoccupied with the desired outcome; you have to be thinking about the process that will lead to that outcome, such as where to focus your eyes. You must be present — not thinking about how big the moment is but asking, ‘What should I pay attention to?’ That approach has to be practiced in low-pressure situations so that it becomes a habit. You have to practice your mindset as well as your movements.”
Julia Colangelo ’12SW, a mindset coach and founder of Hello Flow, a consulting and educational company based in Maui, sees in Larkin’s momentous at-bat a classic example of “flow,” a state of consciousness named by the Hungarian-American psychologist Mihaly Csikszentmihalyi to describe the sensation of being so completely immersed in a task that nothing else seems to exist. Colangelo teaches that flow can be generated through practiced preparatory behaviors — in Larkin’s case, doing stretches and swinging the bat. “By setting up the proper conditions, Larkin made himself available for flow if the opportunity presented itself,” she says.
Research has shown that during the flow state, the prefrontal cortex — the part of the brain associated with complex planning and decision-making — shuts down. “That’s why Larkin, when he got to the plate, entered a flow state,” Colangelo says. “He was prepared: he knew what was being asked of him and what was needed. Once the flow state was triggered, his prefrontal cortex shut off, and when that happens you no longer have self-doubt, inhibition, or fear. You can perform at your highest level without being self-conscious or worried. You radically trust yourself. You can train your brain to do this with repetition and preparation.”
Larkin’s own self-assessment confirms this. “As a full-time role player for most of my major-league career, I took pride in being as best prepared as possible, physically and mentally, before I got in the batter’s box,” he says. “It’s all about preparation.”
Often, Larkin looks back on The Hit and tries to analyze his abrupt shift at the plate from wild anxiety to a pure, laser-focused serenity.
“The only thing I can put together is that I didn’t think Peña could strike me out — I just didn’t,” he says. “I just had to put the ball over the outfielder’s head or hit a ground ball between the infielders, so believing he couldn’t strike me out gave me a sense of calm.
“The bottom line is, I believed I could get the job done, and I was fortunate enough to get the right pitch at the right moment.”
More than thirty years after Larkin’s World Series heroics, the three key drivers of that event survive. The bat he used is displayed on his living-room mantel. The ball rests in a case in Cooperstown, New York, in the Baseball Hall of Fame. And the most important one — his brain — still lights up at the memory of the pitch coming toward him.
To learn more about the brain science of baseball, watch this video from Columbia's Zuckerman Institute: