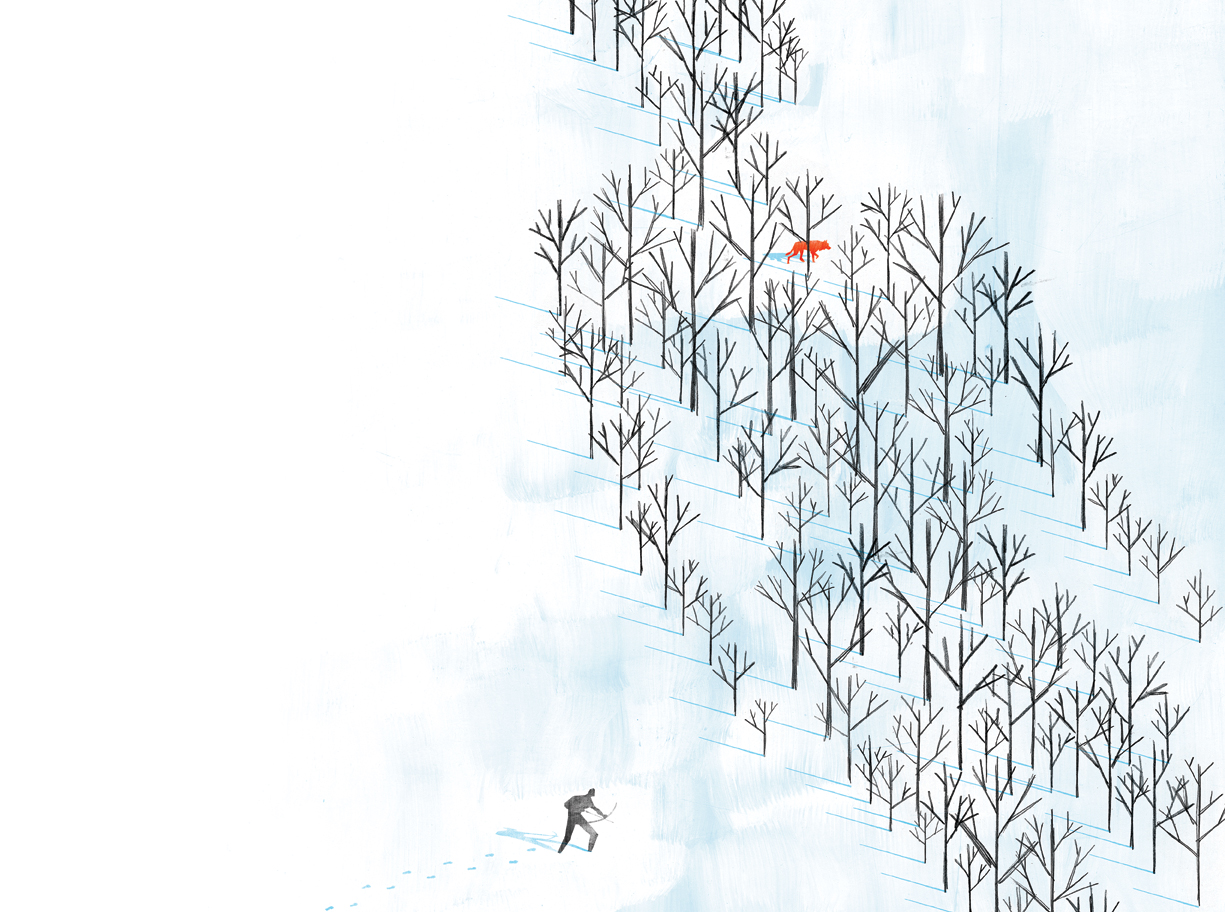
“My doctors are trying to poison me.”
These are the words not of a paranoid schizophrenic but of a clear-minded journalist named Leroy Sievers. Five years after being diagnosed with colon cancer, Sievers, increasingly aware that he would not survive the disease, began documenting his experience in a National Public Radio blog called My Cancer. In it, he related his struggles with the disease’s often-debilitating treatment.
“They call the process chemotherapy,” he wrote in 2006, two years before his death. “The idea is to poison the body enough to kill the cancer but not quite kill the patient. Best I can tell, it’s a difficult line to walk.”
Sievers was discovering what cancer researchers have known for decades: that chemotherapy, despite saving millions of lives since it was created in the 1940s, is a blunt, destructive tool. This is because it attacks all rapidly dividing cells in the body. Cancer cells are rapid dividers and highly susceptible to chemotherapy drugs, but so too are hair cells, skin cells, white blood cells, and cells that compose saliva and stomach fluids. The result is a macabre race to treat the cancer before the drugs destroy the patient.
So why are we still using chemotherapy?
Brent Stockwell, an associate professor of biological sciences and chemistry at Columbia, says it’s because researchers have found it extremely difficult to develop drugs that target cancer cells specifically. Although scientists have made dramatic advances in their understanding of cancer’s basic mechanisms during the past few decades, most cancer drugs being used today are forms of chemotherapy developed as far back as the 1980s.
“There are a handful of so-called ‘targeted therapies’ that have been developed for specific types of cancer, including some forms of leukemia as well as skin, prostate, and breast cancers,” says Stockwell. “The survival rates for these cancers today are quite good. But most forms of cancer are still very difficult to treat because we don’t have therapies that are specific to them.”
To understand why, it is helpful to consider how drugs work. Often, a drug is a tiny molecule that slips inside a cell, attaches itself to a harmful protein, and, in doing so, disarms that protein. But the proteins that are responsible for most forms of cancer are slippery and oddly shaped; drugs have trouble attaching to them. For this reason, as Stockwell explains in his new book, The Quest for the Cure: The Science and Stories Behind the Next Generation of Medicines, many cancer researchers are reluctant to devote their energies to developing drugs that would target cancer cells directly. Instead, they are trying to fine-tune more traditional chemotherapies with the hope of at least extending cancer patients’ lives and relieving some of the drugs’ devastating side effects.
“Today, if you want to look for drugs to interact directly with those tricky proteins inside cancer cells, you’ll have a hard time getting any funding to do it,” says Stockwell. “Federal funding agencies haven’t invested a lot of money into this type of research lately because it’s considered a lost cause. Even if you scrape up the money to do this sort of work, a scientist needs to consider, Am I going to stake my career on a long shot?”
In his own laboratory, Stockwell is swinging for the fences. He believes he can stop the slippery cancer proteins. At the center of his strategy is a specific protein called Ras, which is known to cause 20 percent of all cancers but which most of his colleagues long ago dismissed as “undruggable.”
Stockwell concedes that the odds are against him. But to make big discoveries, he says, you need to take big chances.
“To my mind, there are different approaches to science,” he says. “One approach is that you kind of turn the crank, you do what you’re good at, and you move your field forward with incremental advances. My approach is to look for the home runs, to look for the big unsolved questions, and to try to come up with approaches that could really answer those questions and make a huge impact.”
Drowning in the synthetic sea
Stockwell is a slight, fastidious man with neatly parted black hair and thin-rimmed eyeglasses. His office in Columbia’s new Northwest Corner Building, a science and engineering tower at Broadway and 120th Street, is a study in organization, from the tidy rows of water bottles on his shelf to the precisely angled family pictures on his desk.
Stockwell, a forty-year-old Queens native, did his doctoral work in chemistry at Harvard in the late 1990s, an exciting time for drug discovery. After centuries of deriving medicines mainly from natural compounds, chemists were making great progress in synthesizing molecules that might be used as drugs. Companies were manufacturing huge collections of these synthetic molecules, which they sold to drug researchers. The researchers would essentially hurl these molecules at the protein they wanted to target, in hopes that one of the molecules would stick to it.
While working in the lab of the Harvard biochemist Stuart Schreiber, Stockwell started to look for a molecule that could shut down a squiggly, tapeworm-shaped protein named TGF-beta, which causes some tumors. Over the course of eighteen months, he tested sixteen thousand synthetic molecules assembled by commercial labs, but didn’t find a single molecule that showed any promise of affecting TGF-beta.
“So then I thought, OK, it’s probably not something that we can really find a compound to do,” he says. “It’s just an impossible, undruggable target.”
However, to make sure that he had exhausted all possibilities, Stockwell conducted one last experiment. This time, instead of relying on synthetic products, he used a small library of naturally occurring molecules, some two hundred extracts taken from hard-to-find marine sponges. To his amazement, one of these extracts showed a striking capacity to block the effects of TGF-beta. This taught Stockwell something that many other drug researchers were realizing around the same time: the synthetic molecules being churned out by commercial vendors were, for the most part, lousy drug candidates.
“There had been a lot of excitement about synthetic molecules initially because you could get your hands on huge numbers of them, which seemed to improve your odds of finding one that could act as a drug,” he says. “However, they tended to be flat, architecturally simple products. Natural molecules, in comparison, tend to be larger and more complex. Their structural richness, scientists came to realize, makes them better able to attach to other biological entities.”
As thrilling as the discovery was, Stockwell quickly found that natural molecules had their own drawbacks. Most notably, because they cannot be manufactured, there are limited quantities available. The world’s supply of the sponge extract he had identified was exhausted before Stockwell could finish his experiment. To this day, it remains a mystery whether that extract might have helped treat cancer.
“This is one of the reasons that few scientists today are chasing the most elusive proteins,” Stockwell says. “If you don’t find a drug candidate among the cheaply available synthetic molecules, you’re faced with looking at naturally occurring molecules that are much more expensive and in short supply. It’s a pragmatic challenge that has serious repercussions for our ability to develop new cancer treatments.”
Aiming at cancer’s source
From the nearly floor-to-ceiling windows in his new twelfth-floor office, Stockwell can look out on both Chandler Hall and the Sherman Fairchild Center, where he previously maintained separate chemistry and biology labs. The University conceived of the Northwest Corner Building precisely for researchers like Stockwell: those who work outside the normal confines of academic disciplines, at the nexus of chemistry, engineering, biology, and physics.
“Real-world problems are not simply biology problems or chemistry problems,” Stockwell says. “If you want to understand the physical forces that act upon molecules within human cells, or the electrical properties of single molecules, you need to bring together different types of researchers. Targeting an undruggable protein is this type of complex problem.”
Stockwell’s new laboratory is a bright, open space that is designed for collaboration. On one side, the room connects with the laboratory of Virginia Cornish ’91CC, a chemistry professor who creates artificial cellular pathways as a means of studying disease mechanisms.
“Virginia is also at the chemistry-biology interface,” Stockwell says. “So we put in a shared space between our labs.”
In his laboratory, Stockwell is now trying to find a drug to bind to the Ras protein, which is implicated in about 90 percent of all pancreatic cancers and 50 percent of colon and lung cancers. Its job is to regulate cell growth in the body, which it does by toggling between “on” and “off” positions. When Ras is on, it’s instructing cells to divide; when it’s off, it’s telling them to stop. When Ras is healthy, it’s constantly fluctuating between these two positions. But when Ras breaks down — often as a result of a genetic mutation triggered by carcinogens — it tends to get stuck in the “on” position. The result is runaway cell growth, leading to tumors.
If researchers could find a way to shut down mutant Ras proteins, they might prevent some types of cancer. Scientists have long focused on the Ras protein, and many have tried to find a molecule to dismantle it, but, so far, they have produced nothing. To many scientists, Ras is just one more undruggable protein.
Stockwell isn’t so sure. As in his experience with TGF-beta in graduate school, he believes that finding the right molecule to bind with Ras is primarily a matter of working with better molecules. And he has devised a way to design his own high-quality molecular candidates: he uses sophisticated computer models to synthesize molecules that are physically similar to natural molecules previously shown to have cancer-fighting qualities.
“The molecules we’re designing are based on the physical architectures of two naturally occurring molecules, one of which is found in chocolate and soy beans, the other in certain types of fungi,” Stockwell says. “A few years ago, my laboratory discovered that these two molecules will sometimes kill cancer cells that contain mutant Ras. So we’ve been synthesizing millions of compounds that are slight variations of these natural molecules, with the goal of creating a new molecule that’s perfect for latching onto the mutant Ras and disrupting it. This could enable us to treat many types of cancer, with few side effects.”
Although Stockwell’s approach is more strategic than the conventional method of throwing countless low-quality molecules at proteins to see if any will stick, he still believes that finding the right molecule requires testing many of them. The result is an emphasis on both quality and quantity.
“Our initial results are exciting,” says Stockwell. “We’ve identified some drug candidates that have real potential for locking into Ras. We need to do further experiments, but I’m optimistic we’re going to solve this.”
No more cures?
Even if Stockwell were to identify his elusive molecule tomorrow, an approved drug would likely be ten to fifteen years away. That’s how long it typically takes for a pharmaceutical company to turn a molecule into a safe and effective drug.
In the meantime, Stockwell is pursuing other, more modest ways of improving cancer treatment. He recently made a breakthrough: he synthesized a molecule that, although it doesn’t bind to mutant Ras directly, is effective at killing certain types of cancer cells that contain the protein.
“This isn’t quite the home run we’ve been hoping for, because the molecule doesn’t seem to work against all Ras cancers, but one day it could dramatically improve treatments for some cancer patients,” says Stockwell, who reports his discovery in a forthcoming issue of a major academic journal. “We’re hoping that a pharmaceutical company will develop it into an actual drug that can operate in the human body.”
Stockwell believes the implications of his research go well beyond cancer. In The Quest for the Cure, he argues that scientists’ timidity in studying proteins that they fear are undruggable has contributed to a slowdown in drug discovery that has hobbled the entire pharmaceutical industry: the number of new drugs approved by the FDA annually has halved since the mid-1990s. In his own lab, Stockwell is also trying to disarm proteins that lie at the root of neurological diseases such as Huntington’s.
“All of the 20,000 or so drug products that have ever been approved by the U.S. Food and Drug Administration interact with just two percent of the proteins found in human cells,” he writes in The Quest for the Cure. “This means the vast majority of proteins in our cells — many of which, in theory, can modulate disease processes — have never been targeted before with a drug.”
Many scientists, he says, have taken this as evidence that these harmful proteins yet to be penetrated by drugs cannot be stopped. Pharmaceutical companies have also succumbed to this viewpoint, he says, shifting their priorities away from the discovery of new drugs in favor of finding new applications for existing ones.
“Pharmaceutical companies face tough economic questions,” Stockwell says. “Should they spend their money going after proteins considered to be the most elusive? Or should they focus, perhaps, on fine-tuning their existing drugs in order to discover new clinical applications for them or to improve them just enough so that they can be marketed as new products?”
Pfizer chose to market sildenafil citrate, a heart medication, as Viagra when the company realized that it could be used to treat erectile dysfunction, he points out. And many drug companies have made incremental advances in cancer treatment by combining therapies in novel ways.
“The thousands of drugs that already exist represented the low-hanging fruit, because they work on proteins in our body that were the first ones scientists were able to decode,” Stockwell says. “The prospect of creating new drugs for the trickier proteins is exciting, but it’s also incredibly time-consuming, expensive, and risky. So pharmaceutical companies are choosing to spend a lot of their resources tweaking and repurposing drugs in order to squeeze as much profit out of them as possible.”
This has occurred at the same time that federal funding agencies have gradually shifted their support away from exploratory science endeavors and toward “translational research” that aims to turn proven scientific concepts into real-world tools. “Federal funding agencies really should be stepping in to fund the more basic research that drug companies are backing away from,” says G. Michael Purdy, the University’s executive vice president for research. “But we shouldn’t let the drug companies off the hook. They’re choosing short-term profits over their own long-term financial health and medical progress. That’s going to come back and bite them.”
Luckily, some federal funding for unconventional projects exists. This fall, the National Institutes of Health awarded Stockwell its new EUREKA grant, for scientists conducting “innovative research on novel hypotheses or difficult problems.” The $1 million grant will support Stockwell’s search for a molecule that could bind with the Ras protein.
Stockwell hopes that if he’s successful, his work will inspire other scientists — and funding agencies — to reopen the books on similarly dismissed proteins.
“In the course of time, an increasing percentage of proteins will likely be considered druggable,” he writes in The Quest for the Cure. “The day may even come, far into the future, when all disease-modifying proteins have been targeted successfully with drugs. This will represent the final triumph of human ingenuity, as we reach the pinnacle of medicine — when the majority of possible cures will have been realized.”
It’s a grand description of a utopian future. Which is exactly the point, Stockwell says.
“The book and the research are really about the question rather than the answer,” he says. “There’s no magic bullet that’s going to fix all of these proteins that cause disease, but we need to go after them a lot more aggressively.”