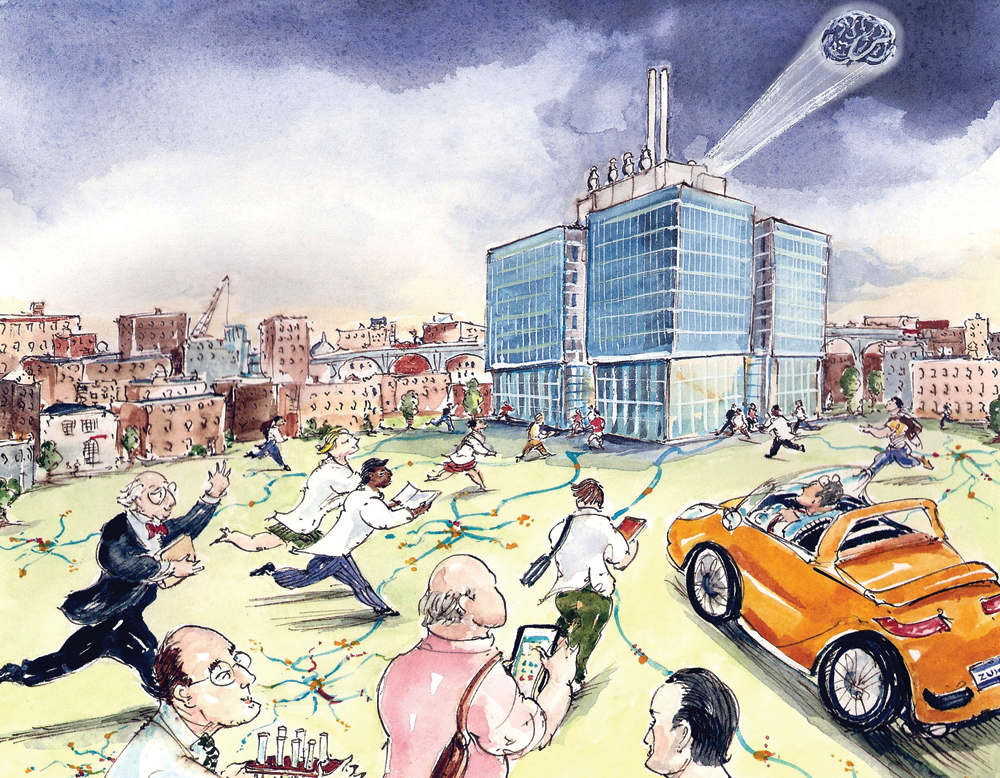
Michael Shadlen is the latest to arrive. He has come from the University of Washington, after running his own laboratory there for seventeen years. Over the summer he packed up his lab equipment, put his family’s Seattle home on the market, and moved to New York City. He has come for a job in a Columbia research program that is still taking shape, in a new laboratory that won’t exist until 2016.
“It’s no fun from a logistical standpoint, because my team will have to move twice,” says Shadlen, one of the world’s top neuroscientists. “But I knew from the first phone call that I wanted in.”
He is in very good company. Larry Abbott, a prominent theoretical neuroscientist who is a wizard at analyzing enormous data sets, came to Columbia from Brandeis in 2005. Stefano Fusi, another leading theoretician, was recruited from ETH Zürich in 2007. Charles Zuker, a biochemist who discovered how the brain perceives taste, arrived from the University of California, San Diego, in 2009. Tom Maniatis, a pioneer of genetic cloning who studies how DNA guides brain development, was plucked from Harvard the next year. Mark Churchland, a young theoretical neuroscientist who has shown that groups of brain cells will sometimes work together like members of a musical ensemble, tapping out rhythmic signals that are meaningful only when combined, left Stanford for Columbia in 2011.
What has drawn them here is the Columbia University Mind, Brain, and Behavior Initiative (MBBI), a new program intended to solve mysteries such as these: How do the electrical impulses of brain cells turn into thoughts? How do these thoughts give rise to all manner of human experience — love, compassion, anger, jealousy?
“The truth is that we are just beginning to study how the brain functions at that level,” says Eric Kandel, an eminent Columbia neuroscientist and one of MBBI’s three codirectors.
Today, most of what is known about the brain involves the molecular composition and functioning of its individual cells, or neurons. The decoding of the human genome has enabled scientists to look deep inside brain cells and identify molecules involved in Alzheimer’s disease and some addictive disorders. At the gross anatomical level, researchers are using new scanning technology to identify areas of the brain where emotions get processed, which may one day help doctors diagnose and treat mood disorders like depression. But ask any neuroscientist how the hundred billion neurons in your brain coordinate their activities to help you read, speak, or decide what to eat, and you’ll likely get a shrug.
“Addressing these questions is going to be the greatest intellectual challenge of the twenty-first century,” says Kandel. “It will have a huge benefit, not only for improving disease treatments, but also for advancing our knowledge of human nature.”
Kandel and fellow codirectors Thomas Jessell and Richard Axel ’67CC have in recent years overseen the hiring of more than a dozen prominent brain scientists. In the next few years, they hope to hire twenty more. Among the enticements they are offering recruits is laboratory space in an elegant new glass building, the Jerome L. Greene Science Center, which is scheduled to open in 2016 on Columbia’s new seventeen-acre campus in Manhattanville. What is unusual about their recruitment effort is that they are not only trying to lure the world’s best neuroscientists to their program, but also physicists, mathematicians, astronomers, engineers, biologists, and psychologists — anybody with fresh ideas to bring to the table.
Roots of contemplation
Shadlen, a youthful fifty-three-year-old with a broad smile, is disarmingly gentle-natured. Like many neuroscientists today, he is fluent in a variety of disciplines — applied mathematics, computer science, physics, and electrical engineering, to name a few — but his broad interests seem to have brought him to the field, rather than the other way around. He was inspired to study the brain, he says, by reading French philosopher Maurice Merleau-Ponty’s writings on perception. He was twenty-two when he published his first paper, in the New Physician, an account of the troubling conditions he had witnessed that spring in a camp for El Salvadorian refugees in Honduras.
“Back in school, I thought I’d wind up smuggling medical supplies to rebels in the hills of South America one day,” says Shadlen, now a professor of neuroscience at the College of Physicians and Surgeons. “But along the way I got distracted by more cerebral concerns, like, how the heck does this gray blob between my ears make me who I am?”
That curiosity has brought Shadlen to one of the most vexing questions in brain science: how do neurons talk to each other by varying the rates at which they fire electrical signals? To keep this problem as manageable as possible, Shadlen has restricted himself over the past two decades to studying a small group of neurons that serve as a way station for visual data, in hopes of making sense of the mathematical language they speak.
“Neurons talk to each other in groups,” he says. “And every member of a group is saying something based on the signals it has received from thousands of other neurons. It’s quite complicated.”
To isolate one set of messages, Shadlen monitors the brain activity of subjects performing cognitive tasks, like identifying which way clusters of dots are moving on a computer screen. After recording the neurons’ firing rates, he then analyzes the data with software, like a cryptographer trying to decipher a strange new code.
Several of Shadlen’s breakthroughs trace back to an observation that he and his postdoctoral adviser, Stanford’s William Newsome, made together in 1994: that neurons in a part of the brain called the association cortex, which is where sensory information gets integrated into our thoughts, are capable of assessing information for several seconds before reacting to it. “A neuron that simply delivers information into your brain or carries motor instructions back out will pass along its message more or less immediately,” says Shadlen. “But we saw that the neurons involved in forming your thoughts would wait for a second or two first, as if they were evaluating the information they had received.”
Shadlen has since shown that neurons in this part of the brain do evaluate the information they receive, in order to determine the data’s significance to thoughts you are experiencing at the moment. To do so, they use a mathematical tool called a “diffusion-to-bound” form of sequential analysis. Here’s how it works: if you were asked which way dots were moving on a computer screen — say, to the right or to the left — neurons in your association cortex would assess the firing rates of other groups of neurons that are sensitive to rightward and to leftward motion. And they would take their time doing the comparison, gathering at least a few milliseconds’ worth of data before calling the contest, to guard against error. Only when the difference between the firing rates of the two groups of neurons hits a precise statistical threshold would your brain form a conscious thought, such as, those dots are moving to the left.
“It is awe-inspiring to see this happen in the laboratory,” says Shadlen. “I believe this is nothing less than the roots of contemplation, of deliberation, of thought itself. These brain cells are exhibiting what I call a freedom from immediacy, in the sense that, rather than responding reflexively to information, they’re ruminating on it.”
Shadlen suspects that this mechanism operates in the brains of most animals, apart from those with the very simplest nervous systems. He hypothesizes that the “neural time integrator,” as he calls it, is duplicated throughout the brains of highly evolved organisms, even in areas responsible for complex functions like memory, language, emotion, and reason. “When this mechanism gets repeated, in layer upon layer of circuitry,” he says, “it eventually produces all different forms of cognition.”
Shadlen doesn’t know this for sure. The only neurons he has observed doing math are those involved in perceiving visual information. That’s because scientists who came before him were able to map the brain circuits for vision in unusual detail, making these circuits the easiest in the brain to monitor. Shadlen has devised clever ways to work around this limitation, though, such as by conducting experiments in which subjects play visual games that require them to keep a running tally of the likelihood that picking certain colors or shapes will bring rewards; the brain circuits for vision thus are made a testing ground for probabilistic reasoning.
“You can see the brain cells updating the probabilities over time, which strongly suggests that this phenomenon isn’t relegated to simple perception, but is a substrate for cognition more generally,” he says.
The idea that the brain uses statistical algorithms to formulate thoughts is not new. The English mathematician Thomas Bayes suggested as much 250 years ago, and scientists and philosophers have since wheeled out the notion periodically. Until now, though, no scientist had ever provided solid evidence for how it might work. Shadlen is regarded by his colleagues as the first to do so, and his work raises a classic philosophical question related to Descartes’s mind-body problem: if scientists can show that our thoughts are the result of pure computation, does this mean we lack free will?
Shadlen is clearly moved by this question, and he has written several papers aimed at philosophers to help them explore the implications of his work. In an essay published in Frontiers in Neuroscience in April, Shadlen and coauthor Adina Roskies, a Dartmouth philosopher, argue that his findings ought not diminish our sense of free will or moral responsibility. For starters, they say, scientists are still a long way from explaining how our most distinctly human traits, like self-awareness and moral thought, come about.
“Like nearly all neuroscientists, we accept physicalism,” they write. “All matters mental are caused by brains. But we leave open the possibility of emergent phenomena: properties that arise from simpler causes but which are not explained away by them.”
In his laboratory, meanwhile, Shadlen is trying to determine if the integrator may ever break down and what the ramifications are. He suspects that this happens quite commonly and that it contributes to a range of disorders that include schizophrenia, Alzheimer’s, and some forms of autism.
“Every neurologist has seen the symptoms, which include a general state of confusion,” says Shadlen. “I think it’s related to the deterioration of the integrator.”
Shadlen came to Columbia, he says, to collaborate with other top neuroscientists who might help him answer questions such as these: What would cause a person’s integrator to break? Are there genetic defects or chemical imbalances involved? Is this mechanism controlled by one part of the brain? Or is it, like consciousness itself, a phenomenon that involves the interaction of lots of brain areas? Can a broken integrator be fixed?
“I need to work alongside people who study the brain at its genetic, molecular, and cellular levels to make sure the principles my team has discovered find their way to the bedside,” he says. “That’s why I’m here.”
Laws of attraction
Columbia’s neuroscience program has always been strong. For many years, however, its reputation was defined by a small number of scientists, including Eric Kandel, eighty-two, a Nobel Prize winner whose research on memory and learning made him, in the eyes of many people, the most important neuroscientist of the twentieth century; Richard Axel, sixty-six, who won a Nobel Prize in 2004 for decoding how the brain perceives smell; and Tom Jessell, sixty-one, a Kavli Prize winner whose work on the assembly of motor-neuron circuits is now a cornerstone of almost all efforts to find stem-cell treatments for neurodegenerative diseases like Lou Gehrig’s.
The program started to expand in the late 1990s. “It had become obvious we had some gaps to fill,” says Jessell, “and the University started to invest in making ours a more well-rounded community.”
The first area that Jessell and his colleagues sought to strengthen was cognitive neuroscience, which involves the study of how our most complex thoughts are rooted in brain physiology. This is the type of work that Shadlen does, and his arrival here was preceded by the recruitment of two other leading cognitive neuroscientists, Mickey Goldberg and Daniel Salzman, in 2001. Both are moving to the Greene Science Center.
The next step was to hire some theoretical neuroscientists. Often trained as physicists, mathematicians, or computer scientists, these expert number crunchers build computer models to predict how brain circuits will behave in different circumstances. In doing so, they are able to help other neuroscientists design appropriate experiments and interpret their data. This is especially helpful to the cognitive neuroscientists, whose work involves analyzing the interactions among neurons in the brain’s most complicated regions, such as the association cortex. In this part of the brain, circuits carrying information related to our senses, memories, and emotions all interact and feed back against each other, making any data collected there very difficult to parse.
Shadlen says the prospect of collaborating with Columbia theoreticians Larry Abbott and Stefano Fusi was a major reason he came here. Abbott and Fusi today are developing ways to pick out signals from some of the noisiest data sets, including those drawn from brain circuits that, in a truly bewildering feat called multiplexing, are able to transmit two messages simultaneously.
“So far, I’ve been able to weed through this stuff,” says Shadlen. “But eventually I want to do experiments where I’m looking at numerous brain circuits as they interact so that I can see how a subject’s thoughts about visual data are being influenced by, say, memories or auditory information. That’s where I’m really going to need those guys.”
Let’s think bigger
It became apparent that the University would need to build a new home for its growing neuroscience program more than ten years ago. The original idea was to create a rather small center on the medical campus, a home only for “card-carrying neuroscientists,” as Jessell puts it. But after Lee C. Bollinger became president in 2002, the plan evolved into something much grander. Jessell recalls being invited, along with Kandel, to Bollinger’s home for breakfast a couple of years later, and listening to Bollinger describe his vision for making brain research an intellectual lynchpin for Columbia’s twenty-first-century campus.
“Now, rather than simply building a new science facility, we’re talking about creating a space for some truly exciting interdisciplinary work, where neuroscientists would be able to interact not just with other scientists but with people in the humanities, business, and the arts, you name it,” says Jessell. “It made sense that this should happen closer to the Morningside campus.”
Plans for the Greene Science Center were publicly announced — along with a lead gift of $250 million from the late Dawn Greene and the Jerome L. Greene Foundation — in March 2006. Renzo Piano, an Italian architect known for his ingenuity in letting natural light into buildings, was commissioned to design a facility with spacious interiors and meeting areas to promote interactions among the one thousand people who will be working there. The building’s construction is now well underway, its gigantic steel columns rising from the ground on a plot where a parking lot, a gas station, and a warehouse once stood.
“Neuroscientists want to have lots of other people around them, because our work is so complicated we can’t possibly solve every challenge on our own,” says Jessell. “Working with chemists, we could come up with new ways of tracking molecules in the brain. With engineers, we could invent new microscopes. We need psychologists and social scientists, meanwhile, to tell us which questions are the most important to ask about the brain and human behavior.”
When the Greene Science Center is fully occupied, approximately two-thirds of its sixty-five labs will be run by neuroscience faculty, with the remainder being run by professors from departments in the Faculty of Arts and Sciences or the engineering school. A handful of researchers from the Morningside campus are already working on MBBI-related projects, including biomedical engineer and brain-imaging expert Elizabeth Hillman and cognitive psychologists Daphna Shohamy and Sarah Woolley.
The Mind, Brain, and Behavior Initiative will also offer a temporary home for scholars in any field — whether law, art history, economics, or public health — to come and work alongside neuroscientists for stays that could range from a few weeks to a couple of years. This is expected to be a popular sabbatical option for scholars, because neuroscientists today are making rapid progress in describing how brain activity guides human behavior. New brain-scanning technology, for instance, has revealed that people clinically defined as sociopaths tend to have anatomical abnormalities that make them feel less empathy. Legal scholars have taken a keen interest, in part because defense lawyers are now regularly submitting their clients’ brain scans as evidence in trials, arguing that people with these physical traits should be held to different standards of legal and moral accountability.
“Clearly, this is an area where neuroscientists need to be working closely with lawyers,” says Kandel. “We first need to figure out which types of anatomical evidence should be considered valid in the courtroom. Then, as we develop therapies to help sociopaths feel more empathy, courts might consider those options when handing out judgments, especially when dealing with adolescents or teenagers who are still developing psychologically.”
Other new areas for interdisciplinary research include the nascent field of neuroaesthetics, in which art historians and brain scientists are studying how our responses to art and music are rooted in brain physiology; and neuroeconomics, which attempts to understand how people make decisions of all types, including, but not limited to, traditional economic decisions like investing. Neuroeconomics is a particularly vibrant area of research now, since many economists believe that brain science may be able to explain why people frequently act irrationally and against their own best interests.
“Studies have shown that people will sometimes overestimate their own abilities, fail to plan for the future, or rely too much on others to look out for their interests,” says Alessandra Casella, a Columbia economist. “But we have no unified theory to explain why people act in these ways. The hope among economists is that by working with neuroscientists we’ll identify brain mechanisms that reveal why people make good decisions in some circumstances and bad decisions in others. That’s a long way off, but it’s the Holy Grail.”
To make sure the Greene Science Center’s environment is conducive to collaborations of this sort, Jessell says, all the neuroscientists being recruited for the program have one thing in common: they are all intellectually adventurous, expansive thinkers.
“We’re not hiring people who are going to hole themselves up in their laboratories,” he says. “They need to come ready to debate, to engage with people outside their world, and to collaborate on this gigantic canvas we are all staring at together.”
Taste and transcendence
On a July afternoon, Charles Zuker sat slouched in a cushiony leather chair in his office at Columbia’s medical campus. He looked stylish and relaxed, his tall and slender frame draped in a loose-fitting cotton shirt and designer blue jeans, sandals on his feet and a pair of reading glasses atop his head.
“You want to know why I’d leave Southern California, right?” he asks with a wide grin. “I’ll tell you why: because I believe in magic. And when you take the craziest, most wildly ambitious and brilliant minds in neuroscience and stick them all together in one building and let them go nuts, that’s what is going to happen.”
Zuker is MBBI’s most prominent recruit aside from Shadlen, and like his new colleague, he is gregarious, funny, and inclined to discussing big philosophical issues related to the brain. But whereas Shadlen has a touch of the earnest and eager schoolboy, Zuker is all cool, calm, and suntanned charisma. He drives a Porsche Twin Turbo, and if you saw him pulling onto campus, you might guess he was somebody famous you should recognize. Shortly before coming to Columbia from UC –San Diego three years ago, he built himself a waterfront home that appeared on the cover of Dream Homes San Diego magazine.
“It had a pool with an infinity edge, which means the water pours off one side like a waterfall,” says Zuker. “Beyond the pool was the ocean. Incredible sensation, that — swimming over the sea. But it was only a home. My soul had to come here.”
Zuker, fifty-four, was raised in Arica, Chile, the grandson of Polish and Russian Jews who fled Europe before the Holocaust. He entered college at age fifteen and earned his doctorate from MIT at twenty-three. He has since discovered nearly everything that is known about our perception of taste. He’s isolated the genes that encode our taste cells, the receptors upon those cells, the chemical pathways that lead up to the brain, and the specific c brain areas that are responsible for our subjective experiences of flavors. In collaboration with researchers at Harvard and the Monell Chemical Senses Center, Zuker has described these mechanisms for all five basic tastes: sweet, sour, bitter, salty, and “umami,” the savory sensation that comes from eating protein.
“Saltiness we nailed just recently,” says Zuker, whose name is the German word for sugar. “It is the trickiest taste to study, because we respond to salt very differently depending on the amount — a little bit is nice, while a lot is gross.”
Studying taste, Zuker believes, could reveal a lot about the human condition. That’s because taste, among all of our senses, is the only one that we respond to in ways that are largely determined by our genes. That is, we all innately like sweetness and are repulsed by sourness, bitterness, and excessive saltiness.
“Sweet is sustenance and bitter is toxic, and for most of our history the way to stay alive was to trust your tongue,” says Zuker. “By contrast, our responses to different smells are almost entirely socially learned, if you can believe it.”
This raises fascinating questions: Why do we like bitter foods like coffee, beer, and olives? Sour lemons and tamarind? Rotten-tasting cheeses like Limburger and pungent fish dishes like hákarl? Zuker suspects it may be for the same reason we like riding roller coasters, bungee jumping, or swimming next to waterfalls: we get a thrill from doing things that our bodies tell us, instinctively, are bad for us.
“There’s something undeniably powerful going on here,” he says. “What is it? Why do we enjoy living on the edge? Why does it make us feel connected to something larger than ourselves? This is what I’m after: I want to understand how we think about sensation.”
Zuker hopes to collaborate with Shadlen to explore these types of questions. “Today, most of us are still studying the brain’s periphery, which is where sensory signals go in and motor signals come out,” he says. “But Mike is venturing deep into the great in-between area, which is where our conscious thoughts are forming. That’s the real wilderness. Just about everybody wants to team up with him.”
Shadlen, in turn, hopes that by working with scientists who are experts on the brain’s chemical composition, such as Zuker, he will contribute to the development of entirely new types of drug treatments for schizophrenia and other mental illnesses. A shortcoming of nearly all existing medications, he says, is that they alter the levels of chemicals found throughout the brain and therefore result in myriad side effects. A solid understanding of the brain’s neural time integrator, Shadlen says, could reveal drug targets that are more specific to the original source of psychiatric disorders.
Despite all the talk of collaboration, neither Shadlen nor Zuker is expecting MBBI to be a love fest.
“There was a time, five or ten years ago, when I probably would have told you that a lot of the scientists who have just become my Columbia colleagues suffered from a sort of molecular hubris, in that they thought that everything you needed to know about the brain could be learned by studying its smallest constituent parts,” says Shadlen. “There’s still some distance between us. We’ve begun finding some common ground because we all realize that the big challenge now is to bring together our puzzle pieces and figure out how they fit together. But we’ll be having some very candid conversations moving forward. I’m expecting the gloves to come off.”
Zuker, when asked his impressions of Shadlen’s neural time integrator, pauses.
“Am I convinced that Mike is pursuing the right questions?” Zuker says. “I’m not sure. But I’ll tell you this: I believe in him as a scientist. I admire his logic and discipline. And our goals are the same: neither of us is satisfied with proteins, genes, circuits — this stuff. We want consciousness, free will, self-awareness. We want drugs that get at the roots of mental illness rather than glossing over the symptoms. We want it all. The whole damn thing.”