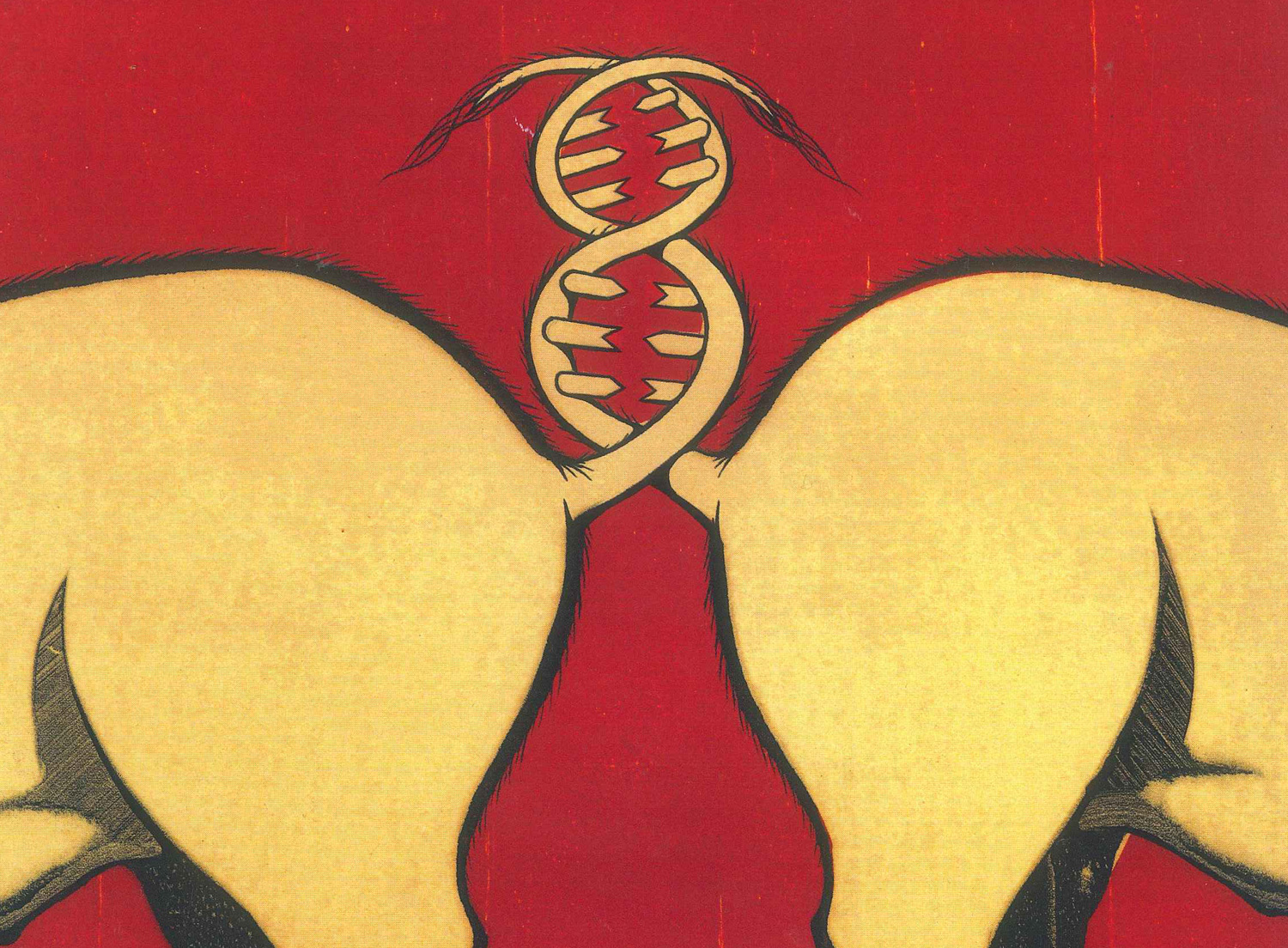
When a new form of influenza appeared in Mexico this past April, Columbia professor Raul Rabadan was in a unique position to identify its origins. Trained originally as a physicist, Rabadan, 35, had spent his early career describing black holes in outer space at the Institute for Advanced Study in Princeton, New Jersey. He had tired of that work about four years ago, though, so he studied biology in his free time and redirected his methods of mathematical analysis toward a poorly understood disease: swine flu.
Until this past spring, most scientists trying to anticipate the next flu pandemic were focused on H5N1 bird flu. That disease has killed nearly 70 percent of its human victims since appearing in Hong Kong in 1997. Fortunately, it doesn’t spread easily among people; virologists and epidemiologists have been monitoring bird flu closely to see if it becomes more contagious.
When Rabadan considered the threat of pandemic influenza, he asked different questions than other scientists. Flu experts, he says, tend to analyze the evolution of viruses on short timescales, in order to detect dangerous genetic changes where and when they occur. “If you’re worried about an outbreak of bird flu,” Rabadan says, “you go out and take samples from ducks to see how that virus is changing, one month to the next. Most people who study diseases work on that scale.” And they typically confine their analyses to small sample sets, he says, in accordance with a custom in the biological sciences that says you should only work on data that you collect.
Rabadan, like many scholars in the emerging field of biomedical informatics, wasn’t interested in obliging this protocol. “I left physics because I saw an enormous amount of biological data being studied only as part of small datasets,” he says. “I thought I could make a difference by looking at the big picture.”
A flu researcher capable of interpreting huge datasets would have a wealth of information at his fingertips, as most grant-giving institutions now require scientists to make public the genetic profiles of any flu viruses that they sequence. Rabadan believed that by sifting through the thousands of long, lettered chains that describe flu virus chromosomes, he could answer wide-screen questions: Does a virus’s propensity to kill a host impart any evolutionary advantage? In what circumstances are dangerous genetic changes most likely to occur? Is it possible to predict a virus’s evolution?
“This led me to focus not on birds,” he says, “but on pigs.”
A sick mix
Pigs play an important role in the evolution of flu viruses and, at least until this spring, an underappreciated one. Among the handful of animals that influenza commonly infects — birds, pigs, dogs, horses, ferrets, whales, and humans — pigs are the most susceptible to catching flu strains from other species. When a pig, or any animal for that matter, becomes infected with two or more influenza strains simultaneously — say, when a farmer gives one of his pigs a human flu at the same time that the animal is fighting a porcine or avian variety — a dangerous new type of influenza can emerge. That’s because two flu viruses in the animal’s respiratory tract can mix genes, creating a new strain that contains enough genetic material from the human flu to infect people and to spread among us, and enough swine or avian flu genes, for instance, to confound our immune system. Such “genetic reassortments” involve much more dramatic alterations than do the routine mutations that occur when RNA strands make mistakes during the self-replication process. Mutations cause flu viruses to evolve constantly in subtle ways, forcing vaccine makers to tweak their drugs every year, while reassortments have spawned the vicious microbes behind all three flu pandemics of the 20th century — those in 1918, 1957, and 1968 — as well as the H5N1 bird flu of current concern. Each of these novel flu strains resulted from a joining of multiple viruses. The mixing vessel in the case of each of the past three pandemics, suspect a growing number of scientists, was the lung of a pig.
When Rabadan was recruited to Columbia from the Institute for Advanced Study last September, as an assistant professor of biomedical informatics, he immediately assembled a team of young number crunchers who could help him explain how flu viruses evolve to become killers. Among his postdoctoral hires are two other physicists, an astronomer, and a computer scientist. Not one of them could dissect a pig — not with a knife, anyway. “We’re all good at math,” says Rabadan, a boyish-looking native of Spain who dresses in designer jeans. “And we study biological phenomena by looking at how genomes change over long periods.”
Last year, Rabadan’s team began analyzing hundreds of swine flu gene sequences, dating back 90 years, held in public databases overseen by the NIH’s National Center for Biotechnology Information. His team looked at the unique genetic blueprints of these viruses against one another, examining how they had mutated and reassorted. Rabadan’s group quickly published some intriguing findings, demonstrating, for instance, that particular genes in swine flu are more likely than others to mutate or to nudge their way into a reassorted virus. Rabadan can’t explain this tendency, but the observation places him on the cutting edge of evolutionary biology. Most scientists in the field study how the physical environment favors some genetic traits over others; the Holy Grail of evolutionary biology has long been to observe — before natural selection obscures the data — the rate at which particular genetic changes occur in the first place. Rabadan is among a number of bioinformaticians who believe that, by analyzing vast quantities of genetic sequences, they can essentially slow down time, identifying patterns in how viruses mutate or reassort before these changes prove adaptable or deleterious, thereby untangling the twin phenomena of gene expression and natural selection.
Birds before swine
Prior to this spring, the practical implications of Rabadan’s work were not obvious. Then on Monday, April 27, 2009, just three days after the World Health Organization (WHO) announced the outbreak of an unfamiliar flu in Mexico, the U.S. Centers for Disease Control and Prevention released the genetic sequences of the new virus samples. Scientists working for the U.S. and Canadian governments had concluded already that the influenza belonged to a family of viruses known as H1N1. They hoped that others could identify its origins and perhaps determine how dangerous it was.
“We dropped everything else we were doing,” says Rabadan. His team first downloaded the genetic sequences of the few dozen flu samples that had been identified by other labs as the novel form of H1N1. They entered these sequences into computer programs, some of which Rabadan’s team had designed, and compared the new H1N1 sequences against the older flu sequences that were publicly available, which included hundreds of swine flu viruses and thousands of human and bird flu viruses.
“The math was actually quite simple in this case,” says Hossein Khiabanian, a postdoctoral researcher in Rabadan’s lab who earned his PhD in astrophysics from Brown. “Basically, we made long lists showing the 13,000 nucleotides of each flu sequence and then determined which viruses shared the largest numbers of nucleotides.” They mapped the new flu strain’s convoluted ancestral lineage this way back several decades. If two viruses matched closely on some of their eight genes but bore little resemblance on other genes, the researchers concluded that one of the two viruses was the product of a genetic reassortment.
“For four days that’s all we did, around the clock,” says Vladimir Trifonov. Another postdoctoral researcher in Rabadan’s lab, he was trained in computer science at the University of Texas at Austin. “It helped that we knew from experience which genes tend to mutate once a virus has jumped from a pig into humans. When we looked at the new samples, we had some idea of what they might have looked like several generations earlier.”
The group’s findings, published the following week in the online journal Eurosurveillance and later in the New England Journal of Medicine, revealed that this new version of H1N1 resulted from a recent genetic reassortment, and an odd one, at that, to have jumped into humans — not a combination of a human flu with a pig flu, but a mix of two flu strains endemic to pigs. Each of the parent strains had infected humans on rare occasions in the past, usually causing mild illness, but neither had been known to spread from person to person.
“That two swine viruses unable to spread between people would combine to form a virus that is contagious is amazing,” says Rabadan. “It would seem to demonstrate the role that chance plays in these events. Influenza is incredibly diverse, which makes it very adaptable and also difficult to anticipate.”
Rabadan’s discovery eventually put to rest debate about what the new illness should be called. Pork industry representatives, soon after the flu outbreak in Mexico was announced, urged scientists, public officials, and journalists to refrain from calling the novel influenza strain “swine flu.” They pointed out that one of the virus’s parent strains itself stemmed from a reassortment that took place among bird, human, and pig influenzas several years ago. They also emphasized that the new virus hadn’t yet been detected in any pigs, only in humans.
Rabadan says it’s true that one of the parent strains contained trace amounts of genetic material from bird and human flus, but that it had been circulating in pigs exclusively for the last 10 years. “If you could follow the ancestral history of any flu virus back far enough, you’d find that it once circulated in other animals,” says Rabadan, adding that all forms of influenza developed originally in birds. “But this new flu, in any meaningful manner of speaking, is from pigs.”
Only a few pigs have been found with the new H1N1 virus, as of this September, but Rabadan insists that’s probably because we’re not looking hard enough. Most countries, including the United States, do not require farmers to test swine for influenza.
“Improving flu surveillance in pigs should be the first lesson we take away from the genetic history of this new influenza,” he says. “For too long we’ve ignored the fact that these animals are a breeding ground for dangerous disease.”
Patient killer?
In the first few weeks of the swine flu outbreak, Mexico reported an unusually high number of flu-related deaths, leading global health officials to fear that they were confronting an illness perhaps as virulent as H5N1 bird flu. New York City shut down several schools, China quarantined travelers from Mexico, and Egypt attempted to slaughter all of its pigs. News media speculated that swine H1N1 might cause a pandemic as deadly as the 1918 Spanish flu, which killed 50 to 100 million people in a catastrophic epilogue to the First World War.
As swine flu spread across the world, though, health officials gradually realized that the vast majority of human illnesses are mild, that the illness is treatable with antiviral drugs like Tamiflu, and that Mexico initially had underreported its total number of nonfatal cases, which made the death rate appear higher than it was. Our bodies, despite swine flu’s posing a novel threat, seem to fight off the illness as easily as they do an ordinary seasonal flu. “Our immune systems are responding to this unfamiliar virus on the fly,” says Rabadan, “and in the time that we’re building antibodies, the virus just doesn’t seem that interested in hurting us.”
However, this flu is unusually contagious, which is to be expected, scientists say, of a virus to which we have no previous exposure. Given its infectiousness, swine flu could rack up a formidable death toll, even in its current mild form. The disease will kill more people this winter than a seasonal flu typically does, WHO predicts, in part because an approved vaccine is not expected to be available before October.
So is there any reason we should be more fearful of catching swine flu than a regular seasonal flu? Is there reason to suspect that it will become more virulent? Yes, says Rabadan: “Swine flu is going through an enormous number of variations in the human body right now, trying to see what works best. Every genetic change is a big roll of the dice.” A seasonal flu, on the other hand, develops a sort of equilibrium with its host. “There’s a history of our immune system fighting off the virus strain,” Rabadan says, “and a history of the virus going through lots of mutations until it figures out a general form that works for its survival.”
Research on other flu pandemics shows that reassorted viruses have become more virulent as they’ve spread. For instance, Stephen S. Morse, a professor of clinical epidemiology at the Mailman School of Public Health, has analyzed clinical data collected in 1918 and determined that the Spanish flu caused a wave of mild illness in New York City months before it started killing millions. Flu viruses may evolve to become more deadly, some scientists have hypothesized, because if they remain in a host’s body for a long time, they increase their chances of infecting others.
“Influenza is always unpredictable,” says Morse, who directs Columbia’s Center for Public Health Preparedness. “But I’ll tell you this: I’d rather catch this swine flu now than later.”
Slippery subject
Rabadan is one of many scientists today parsing the genetic structure of swine H1N1 for clues as to why it has caused mild illness so far. Researchers have discovered that several proteins whose presence is well known to increase the deadliness of influenza are absent in the new swine flu. Most notably, these include the protein PB1-F2, which makes flu viruses especially adept at destroying white blood cells in the nose, lungs, stomach, and intestines. This protein was present in the virus that caused the 1918 Spanish flu and it is present in H5N1 bird flu.
Rabadan and his Columbia colleagues recently examined a century’s worth of flu viruses for the presence of PB1-F2. They demonstrate in a forthcoming paper that the tiny gene section that controls the production of this protein is more likely than others to join a newly reassorted virus. That’s bad news. On a brighter note, Rabadan argues in another forthcoming paper that PB1-F2 serves little useful function. It may frequently appear, he says, only because it is part of a larger gene section that is essential to the flu virus. “This means that when PB1-F2 is broken, as it is in the swine H1N1,” he says, “nature may still favor the proliferation of this mild version of the virus.”
What are the implications of this knowledge? Most of Rabadan’s discoveries, including those involving PB1-F2, are valuable today primarily as basic science, as stepping-stones for research projects that might develop new vaccines or antiviral drugs many years from now. Rabadan acknowledges that he can’t reliably estimate the likelihood that the H1N1 swine flu virus will begin producing PB1-F2, for example. Nor can he predict the likelihood that swine flu will undergo another genetic reassortment, say, with H5N1 bird flu, thereby producing a virus that is both contagious and deadly, or with a flu strain resistant to Tamiflu.
“But that’s my ultimate goal,” Rabadan says. “I believe there are rules to how evolution works and that computational biology is beginning to reveal them.”
Rabadan’s most important discovery toward this end, he says, pertains to all flu viruses that have jumped from animals into humans and circulated among us. His research shows that since 1918 flu viruses in humans have steadily increased their production of the nucleotide uracil. “We believe we can reliably chart future uracil presence in human flu viruses,” says Rabadan, “like a red line pointing upward.” His team is currently working with immunologists at New York University in hopes of explaining this phenomenon; one hypothesis is that uracil helps the virus elude the human immune system.
In the meantime, Rabadan is collaborating with Columbia’s Center for Infection and Immunity, which specializes in identifying unfamiliar microbes. The center is directed by Columbia epidemiologist W. Ian Lipkin, who identified the viruses that cause West Nile Fever and SARS. Lipkin’s lab will genetically sequence swine flu samples for the New York City Department of Health this fall; Rabadan’s lab then will analyze the sequences against large databases of influenza genomes.
“If we get a sample from somebody who’s extremely sick, we can look for genetic clues to explain why the virus has become especially virulent,” says Rabadan. “That could help government labs know what dangerous genetic changes to look for in the future.”
Rabadan also is monitoring how H1N1 swine flu is evolving in various parts of the world. This information could enable vaccine makers to tailor their drugs to different continents.
“The swine flu virus that’s circulating now in Europe is already quite different from the swine flu in South America, which is different from the version in New York, which is different from the version in California,” Rabadan says. “When vaccines finally get administered this winter, we’re going to see some fascinating data. Will the vaccine work in some places and not in others? Which viruses will be able to evade the vaccine? How will they do it? The numbers are going to come alive.”